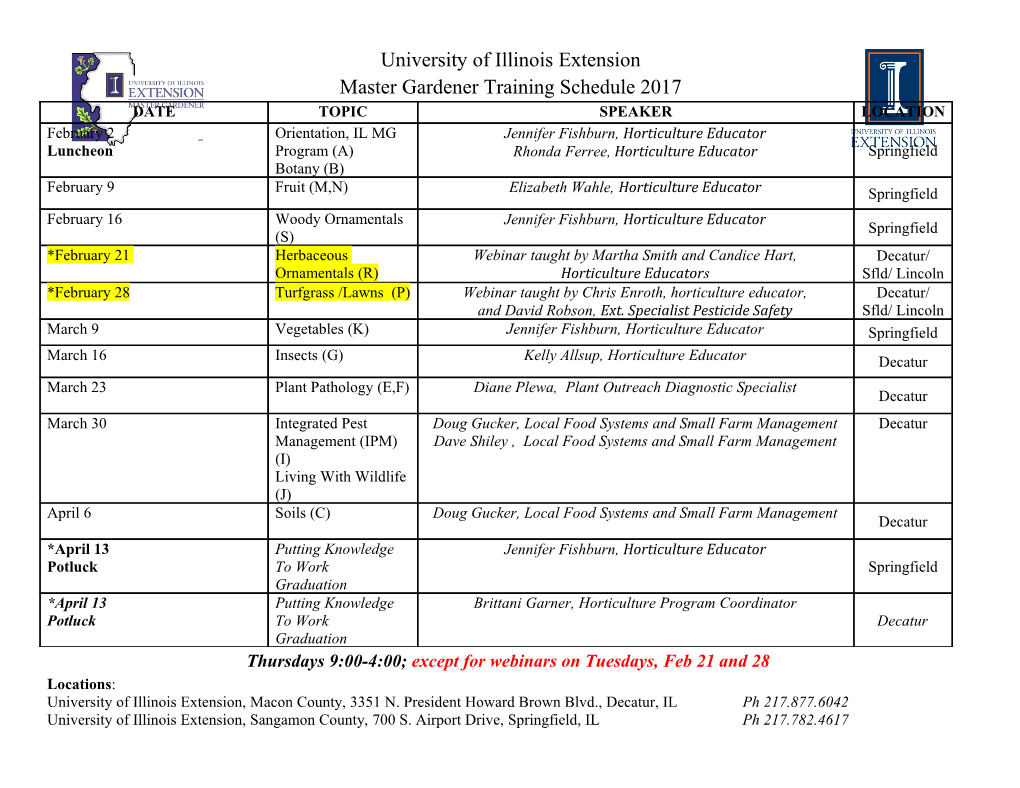
Supplementary information Band alignment and minigaps in monolayer MoS2-graphene van der Waals heterostructures Debora Pierucci1, Hugo Henck1, Jose Avila2, Adrian Balan3,4, Carl H. Naylor3, Gilles Patriarche1,Yannick J. Dappe5, Mathieu G. Silly2, Fausto Sirotti2, A.T Charlie Johnson3, Maria C. Asensio2, and Abdelkarim Ouerghi1,* 1 Centre de Nanosciences et de Nanotechnologies, CNRS, Univ. Paris-Sud, Université Paris-Saclay, C2N – Marcoussis, 91460 Marcoussis, France 2 Synchrotron-SOLEIL, Saint-Aubin, BP48, F91192 Gif sur Yvette Cedex, France 3 Department of Physics and Astronomy, University of Pennsylvania, 209S 33rd Street, Philadelphia, Pennsylvania 19104 6396, USA 4 LICSEN, NIMBE, CEA, CNRS, Université Paris Saclay, CEA Saclay, 91191 Gif-sur-Yvette 5 SPEC, CEA, CNRS, Université Paris Saclay, CEA Saclay, 91191 Gif-sur-Yvette Cedex, France *Corresponding author, E-mail: [email protected], fax number: +33169636006 This Supporting Information includes: Methods and Figures S1 to S7. I. Materials and Methods: Growth of monolayer graphene/4H-SiC(0001): monolayer graphene was produced via a two-step process beginning with a starting substrate of 4H-SiC(0001). Prior to graphitization, the substrate was hydrogen etched (100% H2) at 1550 °C to produce well-ordered atomic terraces of SiC. Subsequently, the SiC sample was heated to 1000 °C and then further heated to 1550 °C in an Ar atmosphere. The sample was cooled down to room temperature and transferred ex-situ to perform different measurements. Growth of MoS2/SiO2/Si(001): MoS2/SiO2 samples were grown by chemical vapor deposition (CVD) in a 1” quartz tube furnace. Microliter droplets of saturated ammonia heptamolybdate solution were dried onto the corners of a Si/SiO2 growth substrate that had previously been coated with a layer of sodium cholate (1% solution spin-coated at 4000 rpm for 60 sec). The growth substrate was placed in the center of the furnace and heated to 800 °C. A 25 mg sulfur pellet was placed on a piece of silicon and positioned upstream in the furnace such that its temperature was approximately 150 °C. Carrier gas (500 sccm N2) was used to bring sulfur vapor into the furnace for a 30 min growth period. Characterization of MoS2/graphene heterostructure: PL measurements were carried out using a commercial confocal Renischow micro-Raman microscope with a 100× objective and a Si detector (detection range up to ~ 2.2 eV). The Raman spectra measurements were performed on the same microscope using a 532 nm laser in an ambient environment at room temperature. The excitation laser (wavelength 532 nm) was focused onto the samples with a spot diameter of ~1 μm and incident power of ~1 mW. The integration time was optimized to obtain a satisfactory signal-to-noise ratio. To analyze the Raman spectra a subtraction procedure has been done. S1 The spectrum of a clean SiC substrate has been used as reference and subtracted from that of the graphene- covered one. XPS/ARPES: XPS experiments were carried out on the TEMPO beamline1 (SOLEIL French synchrotron facility) at room temperature. The photon source was a HU80 Apple II undulator set to deliver linearly polarized light. The photon energy was selected using a high-resolution plane grating monochromator, with a resolving power E/ΔE that can reach 15,000 on the whole energy range (45 - 1500 eV). During the XPS measurements, the photoelectrons were detected at 0° from the sample surface normal 풏⃗⃗ and at 46° from the polarization vector 푬⃗⃗ . The spot size was 100 × 80 (H×V) μm2. A Shirley background was subtracted in all core level spectra. The C 1s spectrum was fitted by a sum of a Gaussian function convoluted with a Doniach-Sunjic lineshape. An asymmetry factor α was used, where α = 0.1 eV (peak G) and α = 0 eV (SiC an IL). The Si 2p, Mo 3d and S 2p spectra were fitted by sums of Voigt curves, i.e, the convolution of a Gaussian (of full-width at half-maximum GW) by a Lorentzian (of full-width at half- maximum LW). The LW was fixed at 45 meV2 for the Si 2p and 90 meV for Mo 3d and S 2p3. ARPES experiments were performed at a photon energy of 100 eV, using linearly polarized light, at a base pressure of 5x10-11 torr and keeping the sample cooled at 40 K. The ANTARES beamline at the SOLEIL laboratory is equipped with a Scienta R4000 hemisperical electron analyzer with a detection system based on a 40 mm diameter multi-channel plate detector. The wide angle lens of the detector, set with an acceptance angle of 25° or 14°, has excellent angular and transmission properties for accurate and fast band mappings. The angle and energy resolutions of the ARPES measurements were set at 0.2° and 10 meV. HAADF-STEM: HAADF-STEM images have been obtained with a Titan Themis 200 microscope equipped with a Cs corrector on the probe and a Super-X detector for the EDX microanalysis The probe current was 120 pA and the convergence half-angle of the probe 24 mrad. The inner and outer (half) angles detection of the annular detector were respectively 70 and 200 mrad. The pixel size for the atomic resolution STEM image (2k x 2k pixels) was 10 pm. References: (1) Polack, F.; Silly, M.; Chauvet, C.; Lagarde, B.; Bergeard, N.; Izquierdo, M.; Chubar, O.; Krizmancic, D.; Ribbens, M.; Duval, J.-P.; Basset, C.; Kubsky, S.; Sirotti, F.; Garrett, R.; Gentle, I.; Nugent, K.; Wilkins, S. AIP Conf. Proc 2010, 1234, 185–188. (2) Bozek, J.; Bancroft, G.; Cutler, J.; Tan, K.; J. D. Bozek, G. M. Bancroft, J. N. Cutler, and K. H. T. Phys. Rev. Lett. 1990, 65, 2757–2760. (3) Mattila, S.; Leiro, J. a.; Heinonen, M.; Laiho, T. Surf. Sci. 2006, 600, 5168–5175. S2 II. Supplementary Figures S1. CVD growth of MoS2 on SiO2 Figure S1: (a) Optical image of CVD grown MoS2 flakes on SiO2, (b) micro-Raman maps of peak intensity of 1 the E2g and A1g modes of MoS2 on SiO2. S2. HR-XPS spectra of pristine graphene underlayer Figure S2: High resolution XPS of pristine graphene: (a) C 1s core level, (b) Si-2p core level. S3 S3. Valence band maximum and Work function measurements: Figure S3: High resolution photoemission study of MoS2/graphene band alignment: (a) The angle integrated photoemission spectra of the valence band for the pristine graphene (cyan curve) and MoS2 graphene heterostructure (blue curve) over the full BZ, measured with h=350 eV (b) Secondary electron cut-off for determining the work function of the MoS2/graphene heterostrucure measured at h=350 eV. S4. Pristine graphene band structure: Figure S4: ARPES spectrum of pristine graphene measured at hν = 100 eV, through the K point, perpendicular to the ΓK direction. S4 S5. EDC curves at the K point of the graphene Brillouin zone: Pristine Graphene MoS /Graphene 2 Intensity (Arb. Units) (Arb. Intensity -2.0 -1.5 -1.0 -0.5 0.0 0.5 Binding Energy (eV) Figure S5: EDC curves at the K point of the graphene BZ for the pristine graphene (black curve) and MoS2/Graphene heterostructure (blue curve). The position of the Dirac point (-0.3 eV), indicated by the black dashed line, does not evolve after the MoS2 deposition indicating that no significant charge transfer from MoS2 to graphene was present. S6. ARPES and EDC curves of MoS2/Graphene heterostructure for different twist angles: Figure S6: Band structure comparison for different twist angles between MoS2 and graphene: a) ARPES spectrum of MoS2/graphene heterostructure without the presence of mini gaps in the π band of graphene, b) Energy distribution curves (EDC) extracted from the ARPES map in a). c) ARPES spectrum of MoS2/graphene heterostructure showing the presence of mini gaps in the π band of graphene, d) EDC curves extracted from the ARPES map in c). The black lines in figure d) show the position of the mini gaps. S5 S7. ARPES and EDC curves of MoS2/Graphene heterostructure, mini gaps width determination: (a) (b) 0.44 ± 0.05 meV (c) 0.36 ± 0.05 meV -3 1 2 3 -4 Intensity (Arb. Units) Intensity (Arb. Units) Intensity Gap 1 Gap 2 4 (eV) -4.8 -4.4 -4.0 -3.6 F -4.4 -4.0 -3.6 -3.2 -5 E-EF (eV) E-EF (eV) E-E 0.33 ± 0.05 meV 0.36 ± 0.05 meV (d) (e) -6 Intensity (Arb. Units) -7 Gap 3 Intensity (Arb. Units) Gap 4 0.75 1.00 1.25 -1 -5.0 -4.8 -4.6 -4.4 -4.2 -6.0 -5.6 -5.2 -4.8 k// (Å ) E-EF (eV) E-EF (eV) Figure S7: Determination of the minigaps width in the graphene π‐band: a) ARPES spectrum of MoS2/graphene hetrostructure. EDC curves across of (b) gap 1, (c) gap 2, (d) gap 3 and (e) gap 4. The EDC curves taken along the green line indicated in (a) for the four different minigaps, show the reduced intensity in the gap region. The minigaps width corresponds to the energy difference between the maximum of the highest binding energy branch and the maximum of the lowest binding energy branch. S6 .
Details
-
File Typepdf
-
Upload Time-
-
Content LanguagesEnglish
-
Upload UserAnonymous/Not logged-in
-
File Pages6 Page
-
File Size-