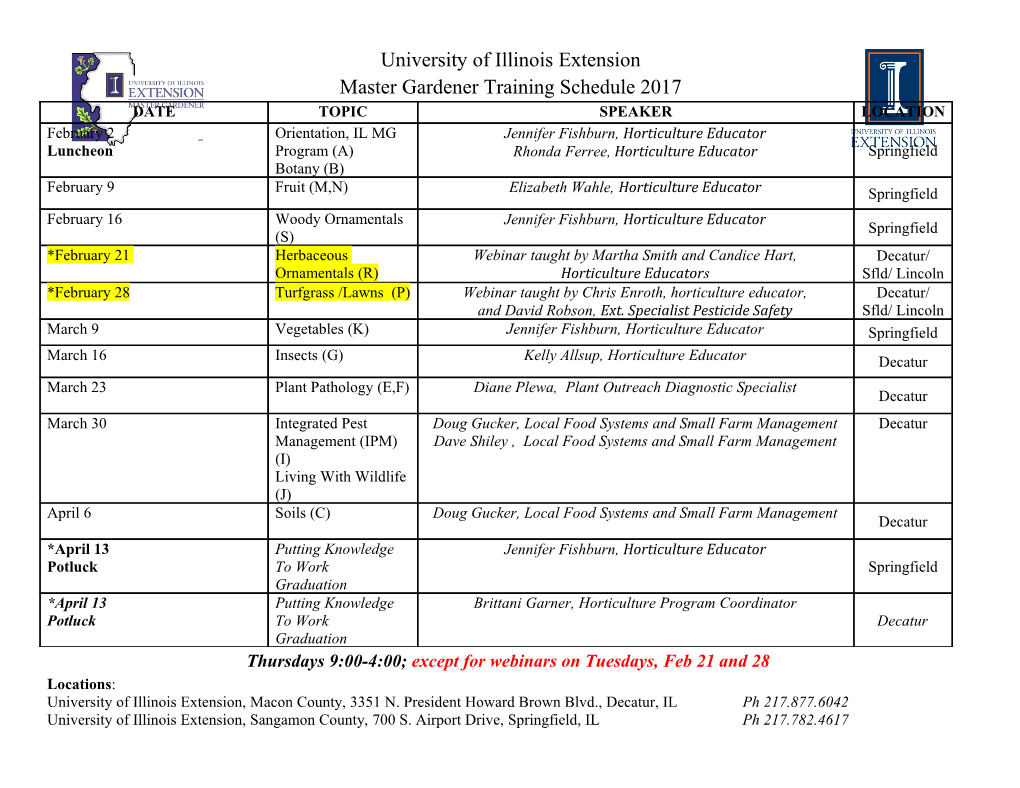
Zinc-binding to leukocyte cell derived chemotaxin 2 and implications for its associated amyloidosis By Jethro Emmanuel Prinston A thesis submitted in conformity with the requirements for the degree of Master of Science Graduate Department of Biochemistry University of Toronto ©Copyright Jethro Emmanuel Prinston, 2020 i Zinc-binding to leukocyte cell derived chemotaxin 2 and implications for its associated amyloidosis Jethro Emmanuel Prinston Master of Science Graduate Department of Biochemistry University of Toronto 2020 Abstract Deposition of leukocyte cell-derived chemotaxin 2 (LECT2) as amyloid (ALECT2) leads to ALECT2 amyloidosis, an amyloid disease with no known cure. Recent findings indicate that ALECT2 amyloidosis is severely underdiagnosed. All sequenced ALECT2 amyloidosis patients have a single nucleotide polymorphism that changes an isoleucine (WT) to a valine (58V) at position 58. Currently, little is known of the mechanism by which the protein misfolds and aggregates. Herein, we demonstrate the first molecular characterization of the misfolding and aggregation of the mature LECT2 protein. Through various biophysical methods, we found that zinc-binding alters the secondary structure, increases the conformational stability, and reduces aggregation propensity of both WT and 58V-LECT2. No significant difference was found between the variants in their secondary structure, conformational stability, and zinc-binding affinity. However, zinc-free 58V-LECT2 was significantly more amyloidogenic than WT-LECT2. Therapies aimed at ALECT2 amyloidosis should exploit putative conformational differences between zinc- bound and zinc-free LECT2. ii Acknowledgements This study would not be made possible without the support of several individuals. I thank current and previous members of the Chakrabartty Lab: Dr Natalie Galant, Meghan Wing, Dr. Kevin Hadley, Dr. Yulong Sun, Joseph Marsilla, and Allison Medina- Cruz for their support and the time spent together. Special thanks to Dr. Natalie Galant for being the life of the lab, for her willingness to help, and for being a role model scientist. I thank Meghan Wing for being the Natalie to my Yulong. I thank Dr. Kevin Hadley for answering my impromptu questions in the lab and for his supportive nods during presentations. I thank Dr. Yulong Sun for his help with experimental design and for performing the site-directed mutagenesis and subsequent cloning for 58V-LECT2. I thank Dr. Avijit Chakrabartty for his supervision and the opportunity to partake in this study. I thank Dr. Simon Sharpe and Dr. Walid Houry for their critical evaluation and insightful discussions. iii Table of Contents Abstract ii Acknowledgements iii Table of Contents iv List of Figures vii List of Tables viii List of Abbreviations ix Chapter 1: Introduction 1 1.1 Background 1.1.1 Protein folding 1 1.1.2 Protein folding and the hydrophobic effect 2 1.1.3 Molecular chaperones in folding protein 4 1.1.4 Protein misfolding and pathology 5 1.2 ALECT2 Amyloidosis 1. 2.1 Pathophysiology of ALECT2 amyloidosis 7 1. 2.2 ALECT2 amyloidosis and its ethnic bias 9 1. 2.3 Diagnosis of ALECT2 amyloidosis 10 1. 2.4 Implications of the I58V change in ALECT2 amyloidosis 10 1. 2.5 Structure and reported physiological activities of LECT2 11 iv 1. 2.6 Potential pathogenesis of ALECT2 amyloidosis 14 1.3 Rationale and Present Study 15 Chapter 2: Materials and Methods 2.1 Protein Expression and Purification 19 2.2 Placement of Disulfide Bonds 20 2.3 Circular Dichroism Spectroscopy 20 2.4 Tryptophan Fluorescence Spectroscopy 21 2.5 Urea Denaturation 21 2.6 LECT2 Zinc Affinity 22 2.7 pH Dependence of Aggregation 23 2.8 Time Course of Aggregation 24 2.9 ThT Fluorescence Assay 24 Chapter 3: Results 3.1 LECT2 is recombinantly expressed with the correct disulfide bonds 26 3.2 Zinc binding alters conformation of LECT2 29 3.3 Zinc binding stabilizes LECT2 33 3.4 WT and 58V-LECT2 have no significant difference in affinity for zinc 37 3.5 Loss of zinc from LECT2 significantly raises its propensity for aggre- gation 39 v 3.6 Zinc-free LECT2 might be the aggregation-prone intermediate that leads to amyloid 42 3.7 Zinc-free 58V-LECT2 forms amyloid, whereas zinc-free WT-LECT2 forms non-amyloid aggregates 44 Chapter 4: Discussion 47 Chapter 5: Future Directions 54 References 57 vi List of Figures Figures Page 1 Ribbon diagram of LECT2. 13 2 Proposed hypothesis of the formation of misfolded LECT2 and sub- 16 sequent amyloid fibrils. 3 SDS-PAGE gel showing different steps of the purification process for recombinant LECT2. 27 4 Schematic representation of the amino acid sequence of the purified recombinant LECT2 protein. 28 5 Far-UV circular dichroism spectra of LECT2. 31 6 Tryptophan fluorescence spectra of LEC2. 32 7 Denaturation of LECT2 monitored by fluorescence. 34 8 Unfolding curves of LECT2 after baseline subtraction. 35 9 Zinc-binding to WT or 58V-LECT2 monitored by fluorescence. 38 10 pH-dependence of aggregation of zinc-bound and zinc-free LECT2. 41 11 Time-course of aggregation of zinc-bound and zinc-free LECT2. 43 12 ThT fluorescence of aggregated WT and 58V-LECT2. 46 vii List of Tables Table Page 1 Thermodynamic parameters governing the denaturation of zinc-bound and zinc-free LECT2 at pH 7.4 36 viii List of Abbreviations A Adenine AD Alzheimer’s disease AL Amyloid light-chain ALECT2 Amyloid leukocyte cell derived chemotaxin 2 ALS Amyotrophic lateral sclerosis ATP Adenosine triphosphate Aβ Amyloid β ATTR Amyloid transthyretin BBB Blood-brain barrier BCA Bicinchoninic acid CD Circular dichroism CNS Central nervous system SOD1 Superoxide dismutase 1 EDTA Ethylenediaminetetraacetic acid ESRD End-stage renal disease G Guanine HD Huntington’s disease I58V Isoleucine to valine change at position 58 IAPP Islet amyloid polypeptide IHC Immunohistochemistry IPTG Isopropyl β-D-1-thiogalactopyranoside Kd Disassociation constant LB Lysogeny broth LCM Laser capture microdissection LECT2 Leukocyte cell-derived chemotaxin 2 MALDI Matrix-assisted laser desorption/ionization MF2 Mag-fura 2 ix mRNA Messenger ribonucleic acid MS Mass spectrometry Ni-NTA Nickel-nitrilotriacetic acid PD Parkinson’s disease PDB Protein data bank RA Rheumatoid arthritis SDS-PAGE Sodium dodecyl sulfate polyacrylamide gel electrophoresis siRNA Small interfering ribonucleic acid SNP Single nucleotide polymorphism ThT Thioflavin T TTR Transthyretin UV Ultraviolet WT Wild type x CHAPTER 1 INTRODUCTION 1 CHAPTER 1 1. INTRODUCTION 1.1 Background 1.1.1 Protein folding Proteins are macromolecules responsible for a multitude of biochemical functions and are central to biological processes in all living systems. A protein’s function is dictated by its three-dimensional structure which is – as postulated by Anfinsen – determined by the protein’s amino acid sequence (Anfinsen, 1973). For a newly synthesized polypeptide to correctly perform its function, it must first fold into its correct three-dimensional structure. This folding process begins as the unfolded polypeptide that is being synthesized by ribosomes forms local segments of secondary structure stabilized by intramolecular hydrogen bonds (Hardesty et al., 2014). Once formed, α-helices and β- sheets, which are often amphipathic, aid in the formation of tertiary structures with polar residues facing the solvent (usually water) and non-polar ones rearranging to form the hydrophobic core of the protein from which water molecules are excluded. Folding is a cooperative process and thus as regions of the protein fold and stabilize its conformation, the subsequent folding of other regions is accelerated. During folding, the polypeptide temporarily assumes various discrete, lower free energy states where it is partially folded and requires some activation energy to proceed to the next state until it reaches the energy levels of the native state. In proteins with multiple domains, folding seems to be hierarchical with smaller regions folding first and interacting to form large regions. The rate at which proteins fold varies greatly due to the large variability in their amino acid 2 sequences and structure patterns. While small proteins can fold in microseconds, larger proteins may require hours to adopt the native state (Ivankov et al., 2009; Naganathan & Muñ Oz, 2005). 1.1.2 Protein folding and the hydrophobic effect Protein folding is a spontaneous process mainly driven by the hydrophobic effect which is the tendency for non-polar substances to associate in aqueous solvents (Southall et al., 2002). The hydrophobic effect facilitates folding by minimizing the number of non- polar residues exposed to water forcing them into the core of the protein (Pace et al., 1996). The hydrophobic effect is to due to the formation of hydration shells comprised of ordered networks of hydrogen bonded water molecules surrounding nonpolar residues. The order of these hydration shells reduces entropy in the system and is therefore thermodynamically unfavourable. During hydrophobic collapse, nonpolar residues fold inward exposing polar ones; this collapse leads to the formation of a molten globule with much of the secondary structure but little tertiary structure. Surrounding water molecules then form hydrogen bonds with the exposed polar residues which reduces both the number of water molecules restricted in the ordered hydration shells and the order in the system. The rise in entropy associated with the decrease of order is favourable and drives protein folding. Once folded, the hydrophobic core stabilizes the protein with the accumulation of abundant van de Waals
Details
-
File Typepdf
-
Upload Time-
-
Content LanguagesEnglish
-
Upload UserAnonymous/Not logged-in
-
File Pages78 Page
-
File Size-