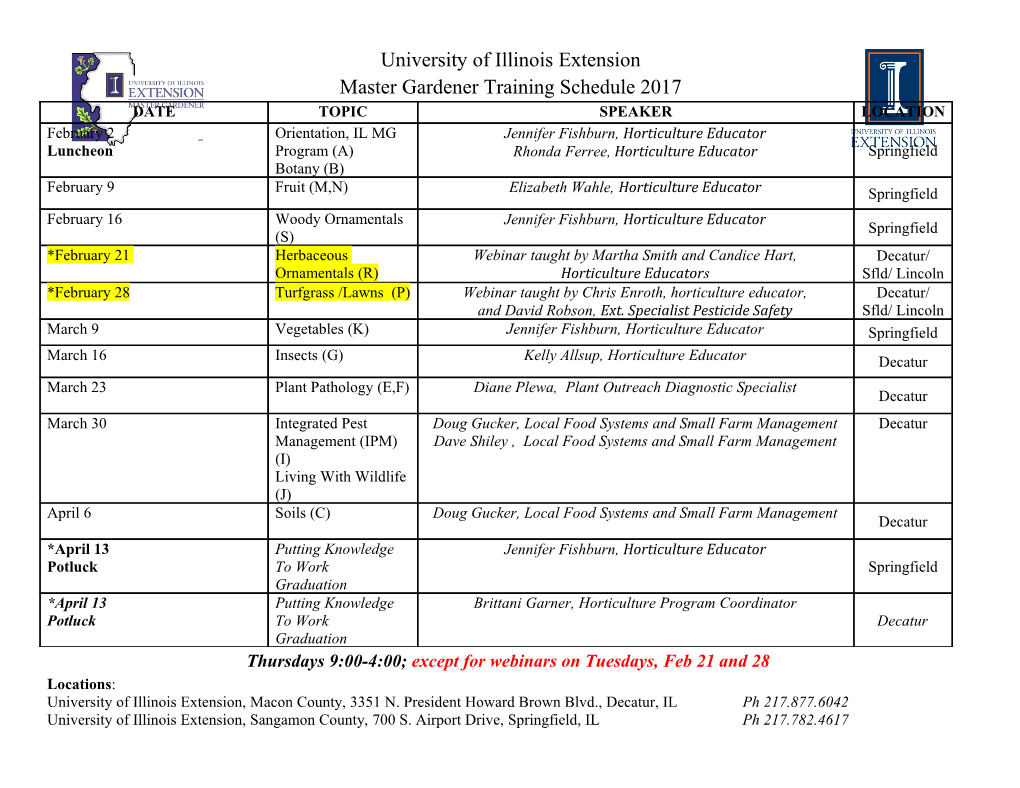
Health Science Campus FINAL APPROVAL OF DISSERTATION Doctor of Philosophy in Biomedical Sciences The Role of Calcium and Mitochondria in the Etiology and Treatment of Three Different Disease Paradigms Submitted by: Christine Brink In partial fulfillment of the requirements for the degree of Doctor of Philosophy in Biomedical Sciences Examination Committee Major Advisor: David Giovannucci, Ph.D. Academic Linda Dokas, Ph.D. Advisory Committee: Joseph Margiotta, Ph.D. Andrew Beavis, Ph.D. Ana Marie Oyarce, Ph.D. L. John Greenfield, M.D., Ph.D. Senior Associate Dean College of Graduate Studies Michael S. Bisesi, Ph.D. Date of Defense: December 7, 2007 The Role of Calcium and Mitochondria in the Etiology and Treatment of Three Different Disease Paradigms Christine A. Brink University of Toledo, Health Science Campus 2007 Acknowledgements First, I would like to thank my advisor, Dr. David Giovannucci, for his encouragement and support throughout my graduate training. His advice and understanding have been essential to my success over the past 5 years. I will always be grateful for the education he has provided to me, and the compassion he has shown toward me. Next, I would like to thank Jenny Giovannucci for her illustrations and encouragement. I would also like to thank Rebecca Pierson for providing the primary cortical neuronal cultures, and countless words of advice. Additionally, I would like to thank Christian Peters for his counsel and assistance throughout our training. In addition, I would like to thank my committee members, Dr. Greenfield, Dr. Dokas, Dr. Margiotta, Dr. Beavis, and Dr. Oyarce, for their contributions and guidance. Lastly, I would like to express my unending gratitude toward my family. My husband’s patience and encouragement have been indispensable during this time. Also, the love and support of my parents were critical in preparing me to take on this incredible and fascinating journey. i Abbreviations Used IP3 1,4,5,triphosphate GABA γ-aminobutyric acid Allo Allopregnanolone AD Alzheimer’s disease Aβ Amyloid-β APP Amyloid precursor protein ALS Amyotrophic lateral sclerosis Ca2+ Calcium CRAC Calcium release activated channel CCE Capacitative calcium entry FCCP Carbonylcyanide-4-(trifluoromethoxy)-phenylhydrazone CAI Carboxyamidotriazole Icrac Current from CRAC channel CIF Cytochrome c efflux inducing factor DMEM Delbeco’s Modified Eagle Medium EC50 Effective concentration at 50% ER Endoplasmic reticulum EDTA Ethylenediamine-tetraacetic acid disodium salt EGTA Ethleneglycol-O, O’ bis(2aminoethyl)N,N,N’,N’-tetraacetic acid GFAP Glial fibrillary acidic protein HVA High voltage activated ii HN Hypothalamic neurohypophysis HPA Hypothalamic pituitary axis ISS Intercellular saline solution LDP Long term depression LTP Long term potentiation LVA Low voltage activated HEDTA N-(2-hydroxyethlenediamine-N, N’, N’-triacetic acid trisodium salt PIP2 Phosphatidylinositol biphosphate PLC Phospholipase C PSS Physiological saline solution PKC Protein kinase C SR Sarcoplasmic reticulum SERCA Sarcoplasmic/endoplasmic reticulum calcium ATPase SCID Severe combined immunodeficency syndrome SNARES Soluble N-ethylmalemide sensitive factor protein receptor SOC Store operated channel TMRM Tetramethyl-rhodamine methyl ester perchlorate TRP Transient receptor protein VGCC Voltage gated calcium channel iii Table of Contents Preliminaries Page Acknowledgments i Abbreviations Page ii Table of Contents iv Chapters Page I Introduction 1 II Methods 6 III Background and Literature Review 15 IV Results 39 V Results 56 VI Results 78 VII Conclusions 103 Bibliography 106 Abstract 139 iv Chapter I: Introduction Calcium (Ca2+) is an important cellular messenger involved in a variety of critical messaging pathways. From cell proliferation to apoptosis, Ca2+ is involved in multiple facets of cell health. Even in the most primitive prokaryotes maintaining the Ca2+ gradient is important, and cytosolic free Ca2+ concentrations are controlled (Case, 2007) . In eukaryotes, Ca2+’s importance is demonstrated early in development, as it controls fertilization and cellular differentiation (Lory et al., 2006). After development, Ca2+ plays a critical role through its control of gene transcription, and is responsible for a myriad of messages including: control of movement, synaptic plasticity, wound healing, insulin secretion and a host of other important functions (Berridge et al., 1998). Consequently, to control all these signaling pathways, Ca2+ uses a diverse range of signaling mechanisms to convey the proper signal for the desired outcome. To achieve specificity of the signals, cells have devised a multitude of means to separate the messages. This is accomplished by a variety of types, locations, amplitudes, kinetics and specific sources of Ca2+ signals. For example, Ca2+ can enter the cytosol through the plasma membrane from a variety of types of Ca2+ channels or transporters, or Ca2+ can be released from internal stores through the action of second messengers like inositol 1,4,5-triphosphate (IP3) on the intracellular release channel, the IP3 Receptor (Broad et al., 1999). Localization of Ca2+ signals is often manifested as microdomains classified by the types of channels involved. Specific examples of these are: sparklets from voltage-operated channels, sparks from ryanodine receptors, blinks which are 1 decreases in ER Ca2+ due to release, and puffs which are responsible for Ca2+ waves induced by IP3 receptors (Berridge, 2006). In addition, there are two types of Ca2+ waves, intracellular and intercellular, and each of these wave types is responsible for different signals (Bootman et al., 2001). There are also a multitude of types of Ca2+ channels that help with specificity of the Ca2+ message. These include: voltage-gated channels (L-type, T-type, R-type, N- type), pumps and exchangers, Ca2+ ATPases, receptor operated channels, second messenger operated channels, and channels on mitochondria and internal stores. Additionally, each of these sources of Ca2+ signaling may be linked to specific physiological outcomes. However, because Ca2+ signaling is so critical and utilizes such diverse pathways, disturbing the critical balance of Ca2+ levels can be devastating to cell health and physiological cell function. Correspondingly, many diseases involve an interruption or unbalance in a Ca2+-signaling pathway. For example, Alzheimer’s Disease (AD), cancer, diabetes, and Parkinson’s disease, have all been linked to a possible deregulation of Ca2+; and in some cases altering the Ca2+ signaling has been considered a possible treatment mechanism. Ca2+ is known to be involved in regulation of the cell cycle. For example, Kao et al (1990) demonstrated that chelating cytosolic Ca2+ blocked the transition from metaphase to anaphase in three different cell types, showing that altering physiological Ca2+ can alter the cell-cycle. Additionally, Guderman and Roelle (2006) found evidence suggesting that disruption of Ca2+ signaling was a key event in the abnormal growth of some small cell lung cancers. Furthermore, 2 many neurodegenerative disorders have focused on Ca2+ dysregulation having a role in the death of neurons. Ca2+ is a major player in the pathways of apoptosis, having a role in both the intrinsic and extrinsic pathways of apoptosis, including those induced by ROS, UV-fragmentation of DNA, and cytotoxic drugs (Hajnoczky et al., 2003). Apoptosis is suspected to play a role in neuronal death in diseases such as AD. Additionally, there are multiple diseases and disorders that involve defects of specific Ca2+ channels. For example, genetic defects in voltage gated Ca2+ channels can result in disorders involving the musculature, neurological, cardiac and vision systems. However, in order to exploit Ca2+ signaling pathways for treatment, a better understanding of the pathways must be established. One important element in understanding Ca2+ signaling is the role of mitochondria. The ability of mitochondria to uptake Ca2+ was first discovered in the 1960’s (Babcock et al., 1997). Mitochondria sequester Ca2+ through the mitochondrial uniporter, a highly selective pore on the mitochondria that utilizes the membrane potential of mitochondria to drive the uptake of positively charged Ca2+ ions (Kirichok et al., 2004). The mitochondrial membrane potential is established through the electron gradient created by the proton-motive force, a mechanism in which positively charged hydrogen ions are moved from inside the inner mitochondrial membrane to outside the membrane. Thus, this process creates a negative charge on the inside of the mitochondrial membrane, which is the driving force for influx of positively charged Ca2+ ions. Therefore, the 3 mitochondrial membrane potential is essential for the maintenance of Ca2+ signaling in mitochondria. Furthermore, mitochondria are intimately involved in shaping many Ca2+ signals (Duchen, 2000; Rizzuto et al., 2000). However, the role of mitochondria in Ca2+ signaling pathways was not fully appreciated until the discovery of Ca2+ microdomains, discussed previously. For example, the role of mitochondria in capacitative Ca2+ entry has recently been identified. When Ca2+ is released from internal stores, store operated channels (SOC) on the plasma membrane are activated, causing them to open and influx more Ca2+. High levels of Ca2+ around the SOC can deactivate the channels, causing them to close. Mitochondria, however, can take up the excess Ca2+ around the SOC keeping the levels low enough for the channel to remain open, allowing
Details
-
File Typepdf
-
Upload Time-
-
Content LanguagesEnglish
-
Upload UserAnonymous/Not logged-in
-
File Pages145 Page
-
File Size-