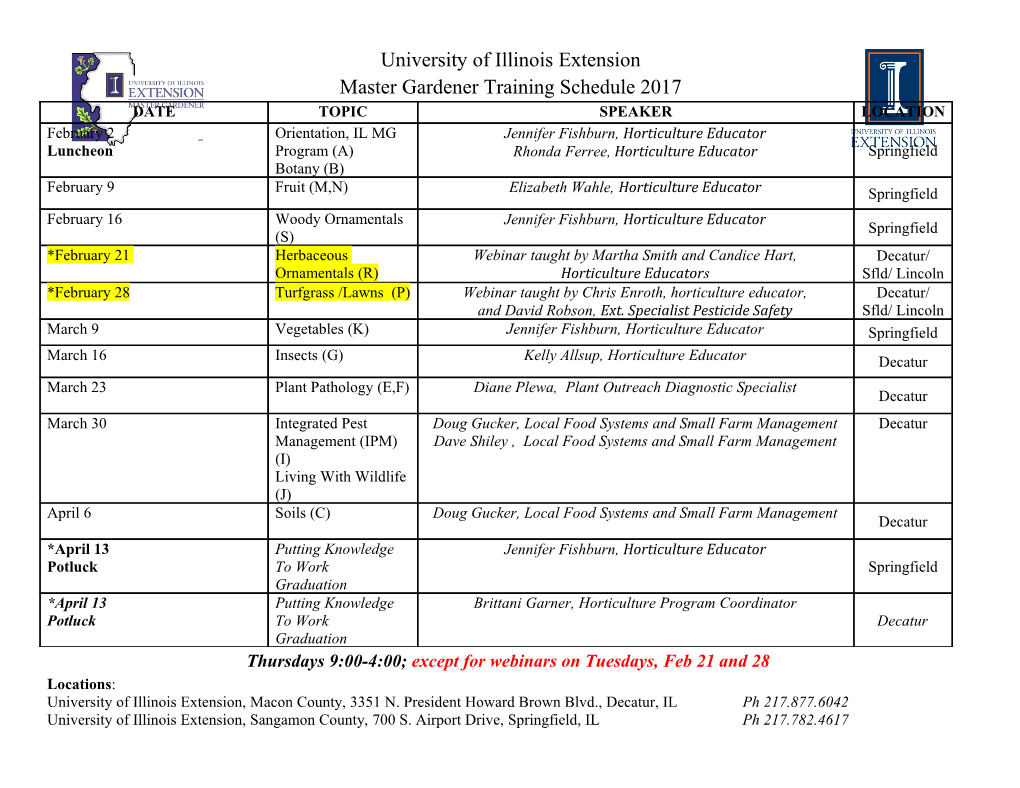
Ecology Letters, (2015) 18: 1234–1241 doi: 10.1111/ele.12503 LETTER Faster diversification on land than sea helps explain global biodiversity patterns among habitats and animal phyla Abstract John J. Wiens* Terrestrial environments occupy ~ 30% of the Earth’s surface yet contain ~ 80% of all species. The causes of this dramatic biodiversity gradient have remained relatively unstudied. Here, I test Department of Ecology and the fundamental prediction that predominantly non-marine clades have more rapid rates of diver- Evolutionary Biology, University of sification than marine clades, using a time-calibrated phylogeny of animal phyla. The results Arizona, Tucson, AZ 85721-0081, strongly support this hypothesis. This pattern helps explain the higher richness of terrestrial envi- USA ronments and the dramatic variation in species richness among animal phyla. The results show *Correspondence: E-mail: wiensj@ the importance of ecology in explaining large-scale patterns of clade richness and of diversification email.arizona.edu rates in explaining Earth’s largest biodiversity patterns. The results also demonstrate remarkable niche conservatism in habitats, in some cases lasting > 800 million years. Finally, the results high- light the surprisingly high species richness of freshwater habitats, which are nearly equal to marine environments despite their much smaller area (~ 2% of Earth’s surface vs. 70% for marine habitats). Keywords Animal phyla, diversification, freshwater, marine, niche conservatism, phylogeny, species richness, terrestrial. Ecology Letters (2015) 18: 1234–1241 (Magallon & Sanderson 2001). Given that animals likely INTRODUCTION originated in oceans long before colonising land (e.g. Jeram The disparity in species richness between marine and non- et al. 1990; Narbonne 2005; Kenrick et al. 2012), higher ter- marine environments is arguably the most dramatic global restrial (or non-marine) richness might be explained by faster biodiversity gradient, but one that remains poorly understood. net diversification rates in terrestrial clades rather than more Estimates suggest that ~ 75–85% of eukaryotic species occur time in terrestrial habitats. However, this hypothesis has not in non-marine (terrestrial and freshwater) environments, yet been tested, at least not at a broad phylogenetic scale. although these habitats occupy only ~ 30% of Earth’s surface Furthermore, some authors have stated that net diversification (May 1994; Mora et al. 2011; Costello et al. 2012). Many rates are uninformative in explaining large-scale diversity pat- authors have commented on this disparity, and have proposed terns (Rabosky et al. 2012). Thus, it remains unclear whether several ecological factors that may be involved (May 1994; higher diversification rates in non-marine clades actually Benton 2001; Vermeij & Grosberg 2010; Grosberg et al. explain higher terrestrial richness. Demonstrating such differ- 2012). These include greater net primary productivity (or ences in rates is necessary before testing more specific greater area of productive habitats) in terrestrial environ- hypotheses as to why they differ. ments, different patterns of primary productivity and her- Here, I test whether occurrence in marine vs. non-marine bivory (typically large plants relative to small herbivores on environments drives higher diversification rates among major land vs. mostly microscopic primary producers in oceans), clades of animals. In doing so, I simultaneously address more difficult movement in water than air, and greater habitat another basic question about global biodiversity: what heterogeneity and geographic isolation on land (reviews in explains the disparity in richness of animal phyla? Animal May 1994; Benton 2001; Vermeij & Grosberg 2010). phyla vary from a single described species (Placozoa) to A more basic hypothesis than any specific ecological factor > 1 million (Arthropoda; Zhang 2013), but few convincing is that higher terrestrial richness is caused by higher diversifi- hypotheses have been proposed to explain these differences. cation rates of predominantly terrestrial clades (e.g. Benton For example, Orme et al. (2002) found that body size did not 2001; Vermeij & Grosberg 2010). Speciation, extinction and explain richness patterns among animal phyla. Given the rich- dispersal are the processes that directly change species num- ness among habitats described above, another hypothesis to bers in a given habitat (e.g. Ricklefs 1987), and ecological fac- consider is that ecology (i.e. marine vs. non-marine habitat) tors must therefore act through these processes to drive explains this disparity, but this has not been explicitly tested. richness patterns. Differences in species richness between habi- To address the hypothesis that non-marine clades have fas- tats should therefore depend on (Wiens 2011): (1) how long ter diversification rates, time-calibrated trees including most each habitat is inhabited (more time for speciation in habitats animal phyla were estimated. Next, the number of described colonised earlier), (2) rates of dispersal between habitats and species in each phylum in each habitat was summarised. Net (3) rates of net diversification in each habitat, where net diver- diversification rates were then estimated for each phylum, sification is the balance of speciation and extinction over time given their age and richness. The hypothesis was then tested © 2015 John Wiley & Sons Ltd/CNRS Letter Marine diversification and global biodiversity 1235 that clades with higher proportions of non-marine species tats, although overall richness patterns have been estimated have higher diversification rates. Major changes in habitat by several authors (e.g. May 1994; Mora et al. 2011; Costello among animal phyla were also reconstructed to help address et al. 2012). Grosberg et al. (2012) summarised information how long animals have been present in each habitat. on species richness in each habitat, but included only 10 ani- mal phyla, and did not provide supporting references. Here, data were assembled on the numbers of extant, described spe- MATERIALS AND METHODS cies in each habitat for all 32 currently recognised extant ani- mal phyla, using literature sources and databases (Table S4). Time-calibrated phylogeny Details are described in Appendix S2. Estimating a new animal phylogeny was not the goal of this Importantly, all estimates of species richness are underesti- study. Instead, existing data were used to estimate a time-cali- mates of actual richness. However, these analyses merely brated tree, and the topology was constrained to reflect well- assume that the proportions of species in different habitats supported relationships found in previous studies. To do this, will remain roughly similar (e.g. more species in terrestrial an expanded version of the matrix of Philippe et al. (2011) than marine habitats), even as more species are discovered was generated. This matrix is modified from that of Dunn and described. This assumption is broadly supported by the et al. (2008), which is relatively comprehensive in its sampling concordance between my estimates based on described species of animal phyla (including 27). This matrix included 150 genes with those incorporating undescribed species (see Results). from 77 taxa (71 metazoans, 6 outgroups) mostly from ribo- somal proteins and ESTs (expressed sequence tags). Philippe Diversification rates et al. (2011) improved upon this dataset by correcting align- ment errors and adding new sequences. To this data matrix, The net rate of diversification for each phylum-level clade was two more putative phyla (Acanthocephala, Placozoa) were estimated using the method-of-moments estimator for stem added here (Tables S1 and S2). Full methods are provided in group ages (Magallon & Sanderson 2001). The stem-group Appendix S1. estimator was used (in part) because the phylogeny included A time-calibrated phylogeny was generated using the Baye- too few species for some phyla to represent their crown group sian relaxed lognormal approach in BEAST, version 2.1.3 age (e.g. some species-poor phyla are represented by single (Bouckaert et al. 2014). Given that analysing the large num- species). More importantly, the stem group incorporates the ber of genes and taxa simultaneously would have been com- entire history of the group (from the first split from its sister putationally challenging, the dataset was reduced to 16 taxon to the present day), whereas the crown group might relatively complete genes. The six non-metazoan outgroups represent only a very recent diversification of extant species were removed. PartitionFinder v1.1.1 (Lanfear et al. 2014) (e.g. a group with a stem age of 200 Ma might have a crown was used to simultaneously find the best-fitting set of parti- age of only 20 Ma, especially if richness was greatly reduced tions and best-fitting model for each partition (Table S3). by extinction). Therefore, the stem-group diversification rate Two sets of topological constraints were used. The first set better represents the overall net diversification of the clade. was based primarily on the tree of Dunn et al. (2014), with Estimating the diversification rate of a clade using the other relationships constrained based on the trees of Dunn method-of-moments estimator requires both clade age and et al. (2008) and Philippe et al. (2011). The tree of Dunn et al. richness as inputs, and an assumed relative extinction rate (2014) provides a summary of animal phylogeny based on many (epsilon, or e). The relative extinction rate is the
Details
-
File Typepdf
-
Upload Time-
-
Content LanguagesEnglish
-
Upload UserAnonymous/Not logged-in
-
File Pages8 Page
-
File Size-