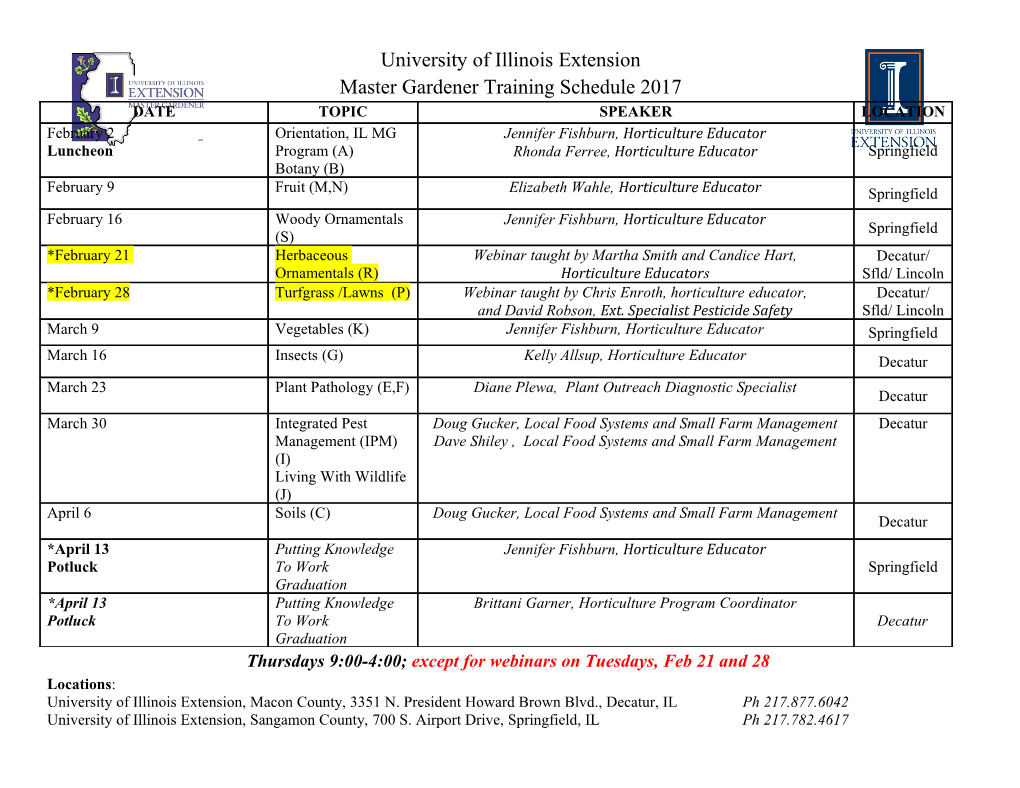
HOW DOES URBANIZATION AFFECT SPATIAL VARIABILITY AND TEMPORAL DYNAMICS OF SOIL ORGANIC CARBON IN THE MOSCOW REGION? Viacheslav I. Vasenev HOW DOES URBANIZATION AFFECT SPATIAL VARIABILITY AND TEMPORAL DYNAMICS OF SOIL ORGANIC CARBON IN THE MOSCOW REGION? Viacheslav I. Vasenev Thesis committee Promotor Prof. Dr R. Leemans Professor of Environmental Systems Analysis Wageningen University Co-promotor Dr J.J. Stoorvogel Associate professor, Soil Geography and Landscape Group Wageningen University Other members Prof. Dr L. Brussaard, Wageningen University Prof. Dr A.J. Veldkamp, University of Twente, Enschede Prof. Dr P.M. van Bodegom, University of Leiden Dr T. Chiti, The Euro-Mediterranean Center on Climate Change, Viterbo, Italy This research was conducted under the auspices of the Graduate School for Socio-Economic and Natural Sciences of the Environment (SENSE) HOW DOES URBANIZATION AFFECT SPATIAL VARIABILITY AND TEMPORAL DYNAMICS OF SOIL ORGANIC CARBON IN THE MOSCOW REGION? Viacheslav I. Vasenev Thesis submitted in fulfillment of the requirements for the degree of doctor at Wageningen University by the authority of the Rector Magnificus Prof. Dr A.P.J. Mol, in the presence of the Thesis Committee appointed by the Academic Board to be defended in public on Tuesday 8 December 2015 at 4 p.m. in the Aula. Viacheslav I. Vasenev How does urbanization affect spatial variability and temporal dynamics of soil organic carbon in the Moscow region? 216 pages. PhD thesis, Wageningen University, Wageningen, NL (2015) With references, with summary in English ISBN 978-94-6257-589-9 Table of Contents 1 General introduction 1 2 Urban soil carbon: What do we know? 17 3 Urban Soil organic carbon and its spatial heterogeneity in comparison to 49 natural and agricultural areas in Moscow region 4 How to map soil organic carbon in higly urbanized regions? 65 5 Analyzing spatial variability of soil respiration by direct and indirect methods 93 6 Basal respiration as a proxy to understand spatial trends in CO2 emissions in 117 the Moscow region 7 Net effect of urbanization on carbon stocks: the case of moscow region 131 8 Synthesis 157 References 171 Summary 205 Acknowledgement 209 About the author 213 SENSE diploma 215 1. GENERAL INTRODUCTION 1.1. Soil organic carbon as a key parameter of soil quality The carbon cycle is one of the principle biogeochemical cycles and likely the most studied one. Carbon stocks in the atmosphere, the ocean and terrestrial ecosystems, and carbon fluxes between them play an important role in key processes, such as soil formation, biomass production and climate change. Carbon stocks and fluxes and their biogeochemical and anthropogenic drivers attract increasing attention of scientitists and policy makers. Several international projects (e.g. the Global Carbon Project, www.globalcarbonproject.org; and the Global Carbon Atlas, www.globalcarbonatlas.org) and intergovernmental initiatives, such as the Intergovernmental Panel on Climate Change (IPCC; www.ipcc.ch) provide global carbon assessments and forecasts. Monitoring networks, such as FLUXNET (www.fluxnet.ornl.gov), and data analysis centers, such as the Carbon Dioxide Information Analysis Center (www.cdiac.esd.ornl.gov) provide timely data on carbon stocks and fluxes for different ecosystems world-wide. The largest pool of actively cycling carbon in terrestrial ecosystems can be found in soils, which accumulates about 1500-2000 Pg (1015 g) C (Swift, 2001; Janzen, 2004). These soil carbon stocks include biochemical substances of different origin (e.g. organic, inorganic and black carbon), chemical structure (e.g. carbohydrates, humic and fulvic acids, and aromatic and aliphatic compounds) and mobility (i.e. active, slow and passive; Coleman et al., 1997; Orlov, 1998; Swift, 2001). Fractioning soil organic carbon is critical to model local carbon turnover. However, in regional and global carbon assessments, where fractional data are limited and the heterogeneity of bioclimatic and soil conditions is large, more integral parameters, such as soil organic carbon (SOC) or soil organic matter (SOM), are used. Historically, SOC and SOM values are widely accepted indicators of soil quality. For example, SOC depletion is used as a basic indicator of soil degradation (Nortcliff, 2002; Bastida et al., 2008). Many local surveys and farmers from all over the world report SOC and SOM content as a critical feature for soil fertility and agricultural quality (Ali, 2003; Barrios and Trejo, 2003; Mairura et al., 2007). Crop models, agroecological models and decision support systems also consider carbon content as an important driver for yield assessments (Rossiter, 1989; 1990; Vasenev and Bukreev, 1994; Albizua et al., 2015). The shift in recent decades from traditional agricultural attitudes of soil as a substrate for food production to its role in essential ecological processes and functions highlighted the importance of soil carbon 1 Chapter 1 stocks and fluxes (Bolin et al., 1979; Kovda & Rozanov, 1988). Carbon sequestration, for example, is an important process to mitigate climate change (IPCC, 2001; Lal, 2003; Janzen, 2004), whereas soil respiration is the largest biogeochemical carbon efflux into the atmosphere, contributing to climate change (Raich et al., 2002; Schulze, 2006). Soil microbial carbon indicates the soil’s performance as a habitat for microorganisms. Soil microbial communities contribute to biodiversity and gene reservoirs (Andrews et al., 2004; Blum, 2005; Dobrovolsky and Nikitin, 2012). The relation between soil microbial carbon and microbial respiration, defines the microbial metabolic coefficient, which is widely accepted as a relevant indicator of the state of microbial soil communities and ecosystem disturbance (Anderson and Domsch, 1985; Dilly et al., 2003; Bastida et al., 2006). Many studies to classify and assess soil functions acknowledge the role of SOC (e.g. BBodSchG, 1998; Karlen et al., 2003; Andrews et al., 2004; Blum, 2005; Dobrovolsky and Nikitin, 2012). Table 1.1 illustrates the major roles that SOC plays for different soil functions from different soil function’s classifications used in the USA, Europe and Russia. Table 1.1 Carbon-related soil functions in different classifications1 Nortcliff et al. Andrews et al. Dobrovolsky et al. Blum (2005) Ritz et al. (2009) BBodSchG (1998) (2002) (2004) (2012) • Biomass production • Provision of • Food and fibre • Participation in • Nutrient • Influence on the • Participating in physical, production water and cycling gas content biogeochemical chemical and • Environmental nutrient cycles • Biodiversity • Storage of cycles, including biological settings interactions, • Decomposition and habitat nutrients gas exchange for living including • Basis for life of • Resistance and • Habitat for between soil and organisms carbon people, plants, resilience terrestrial atmosphere • Supporting retention animals and soil organisms • Source of raw biological activity • Supporting organisms • Source for materials and diversity for habitats and • Land for minerals’ and plant growth biodiversity agriculture and fossils’ • Filtering, sylviculture formation buffering, • An archive of • Transformation degrading organic natural and and transfer of and inorganic cultural history sun energy to substances the earth bowels • Storage of historical artifacts 1 We used the original names of the functions, given by the authors of each classification. Substantial differences in approaches to classify soil functions (in terms of definitions and labels of each function, their total number and the classification’s major purpose) make the comparison in Table 1.1 difficult. For example, the European and American classifications propose six or seven functions, usually subdivided to ecological and non-ecological functions (Blum, 2005) or natural and beneficial-to-humans functions (BBodSchG, 1998) with special 2 General introduction attention to soil–human interactions. In contrast, the Russian classification with 32 soil functions (Dobrovolsky et al., 2012) gives much more attention to the interaction between soils and landscapes (at the biogeocoenotic level) and soils and environments (at regional and global levels). Classifications of soil functions in the UK (Ritz et al., 2009) and Germany (BBodSchG, 1998) were mainly developed to support land-use planning, whereas the Russian (Dobrovolsky et al., 2012) and American (Andrews et al., 2004) classifications are more suited to conserve nature. Although all these approaches differ, they all consider SOC as an important parameter: up to two thirds of the soil functions are directly or indirectly related to SOC stocks. The recently emerged concept of ecosystem services (ESs; MA 2003) expands analyzing environmental properties, processes and functions with human economic benefits (de Groot, 1992; Constanza et al., 1997). Although, soil services are considered part of ESs (Breure et al., 2012), SOC directly or indirectly affect many specific ESs, including soil fertility maintenance, food production and climate regulation (MA, 2003; TEEB, 2010). Information on SOC stocks is widely used in regional and global assessments of soil functions and ecosystem services (Kurbatova et al., 2004; Dominati et al., 2010; Bouma et al., 2015). In this context, the spatial variability and temporal dynamics in SOC play an important role influencing the accuracy of these assessments. The spatial-temporal variability of SOC is influenced by both environmental and anthropogenic factors. Environmental factors, including
Details
-
File Typepdf
-
Upload Time-
-
Content LanguagesEnglish
-
Upload UserAnonymous/Not logged-in
-
File Pages224 Page
-
File Size-