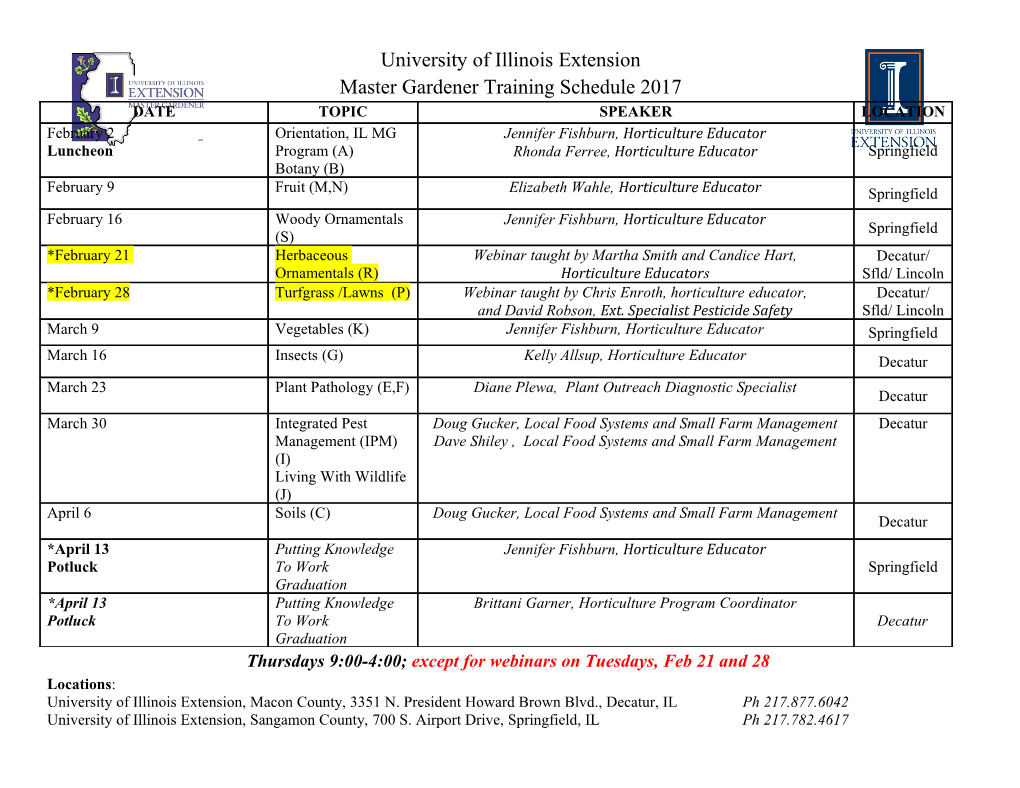
Structural The Space Shuttle—a mostly reusable, human-rated launch vehicle, spacecraft, space habitat, laboratory, re-entry vehicle, and Design aircraft—was an unprecedented structural engineering challenge. The design had to meet several demands, which resulted in innovative Introduction solutions. The vehicle needed to be highly reliable for environments Gail Chapline that could not be simulated on Earth or fully modeled analytically Orbiter Structural Design for combined mechanical and thermal loads. It had to accommodate Thomas Moser payloads that were not defined or characterized. It needed to be weight Glenn Miller efficient by employing a greater use of advanced composite materials, Shuttle Wing Loads—Testing and Modification Led to Greater Capacity and it had to rely on fracture mechanics for design with acceptable Tom Modlin life requirements. It also had to be certified to meet strength and life Innovative Concept for Jackscrews requirements by innovative methods. During the Space Shuttle Prevented Catastrophic Failures John Fraley Program, many such structural design innovations were developed Richard Ring and extended to vehicle processing from flight to flight. Charles Stevenson Ivan Velez Orbiter Structure Qualification Thomas Moser Glenn Miller Space Shuttle Pogo— NASA Eliminates “Bad Vibrations” Tom Modlin Pressure Vessel Experience Scott Forth Glenn Ecord Willard Castner Nozzle Flexible Bearing— Steering the Reusable Solid Rocket Motor Coy Jordan Fracture Control Technology Innovations— From the Space Shuttle Program to Worldwide Use Joachim Beek Royce Forman Glenn Ecord Willard Castner Gwyn Faile Space Shuttle Main Engine Fracture Control Gregory Swanson Katherine Van Hooser 270 Engineering Innovations Orbiter Structural beyond the state of the art were needed. the ascent wing loads were greater The crew compartment had to be than predicted because of the effect Design placed into the airframe such that the the rocket exhaust plume had on the pressurized volume would effectively aerodynamic pressure distribution. NASA faced several challenges in “float.” And it was impractical to As a result, early flights were flown the structural design of the Orbiter. test the full airframe under combined within limited flight regimes to assure These challenges were greater mechanical and thermal loads. that the structural capability of the than those of any previous aircraft, wings was not exceeded. The wings Thousands of analytical design loads launch vehicle, or spacecraft, and the were later “strengthened” with minor and conditions were proven acceptable Orbiter was all three. Yet, the space changes in the design and weight. with flight data with one exception: agency proceeded with tenacity and confidence, and ultimately reached its goals. In fact, 30 years of successful shuttle flights validated the agency’s Shuttle Wing Loads—Testing and unique and innovative approaches, processes, and decisions regarding Modification Led to Greater Capacity characteristics of design. Orbiter wing loads demonstrated the importance of anchoring the prediction or A few of the more significant challenges NASA faced in Orbiter grounding the analysis with flight data in assuring a successful flight. The right wing structural design included the evolution of Columbia was instrumented with strain gauges for the test flights and was of design loads. The Orbiter structure load-calibrated to verify the in-flight air load distribution. The wing was also was designed to an early set of loads instrumented with pressure gauges; however, the number was limited due to and conditions and certified to a later on-board recorder space limitations. This resulted in the need to obtain additional set. The shuttle achieved first-flight readiness through a series of localized pressure data. structural modifications and operational Space Transportation System (STS)-1 (1981) data indicated higher shear in the aft flight constraints. During the early design phase, computer analyses using spar web than was predicted. NASA conducted analyses to determine the location and complex calculations like finite-element magnitude of forces causing this condition. The results indicated an additional load models and techniques for combined along the outboard wing leading edge (elevon hinge line). Data obtained on STS-2 thermal and mechanical loads were not (1981) through STS-4 (1982) substantiated these results. This caused concern for the possible. Later advances in analytical operational wing limits that were to be imposed after the flight test period. methods, coupled with test data, allowed significant reductions in both The additional load caused higher bending and torsion on the wing structure, scope and cost of Orbiter structural exceeding design limits. The flight limits, in terms of angle of attack and sideslip, certification. The space agency had to would have to be restricted with an attendant reduction in performance. face other challenges. Structural efficiency had to be compromised The recovery plan resulted in modification to the wing leading edge fittings. The major to assure versatile payload attachment impact was to the structure between the upper and lower wing skins, which were and payload bay door operations. Skin buckling had to be avoided to graphite-epoxy. These required angle stiffeners on each flat to increase the buckling assure compatibility with the stress. The weight of the modifications resulted in a loss of performance. The resulting low-strength Thermal Protection flight envelope was slightly larger than the original when accounting for the negative System tiles. Composite materials angle-of-attack region of the flight regime. Engineering Innovations 271 Payload Access and Structural Attachments—Mid-Fuselage and Payload Bay Doors Typical Payload Attachment Scheme NASA designed the mid-fuselage of the Orbiter to be “flexible” so as to Primary Fitting accommodate the closing of payload Latch bay doors in space. The design also had Gear Motor to accommodate a wide range of Bridge Pin payload sizes, weights, and number. 3 Payload Sill Longeron The payload bay doors were an integral part of the fuselage structure. The Bridge classical structural design would have Fitting the doors provide strength when the fuselage encountered loads from Primary bending, twisting, shear, internal Fittings X and Z Loads pressure, and thermal gradients. The Stabilizer doors also had to open in space to Fitting Z Loads provide access to the payload and Main Frame enable the radiators to radiate heat to space. Equally important, the doors had Payload Bay Doors to close prior to re-entry into Earth’s atmosphere to provide aerodynamic shape and thermal protection. Keel Fitting To balance the functional and strength Y Loads requirements, engineers designed the doors to be flexible. The flexibility Sets of moveable attachment fittings on the longerons and frames accommodated and zipper-like closing ensured that multiple payloads. The Monte Carlo analyses of the full spectrum of payload quantities, the doors would close in orbit even if distorted thermally or by changes in sizes, mass properties, and locations determined the mid-fuselage design loads. the gravity environment (from Earth These design loads were enveloped based on a combination of 10 million load cases. gravity to microgravity). If the latches Decoupling the design of the mid-fuselage and payloads enabled a timely design of both. did not fully engage, the doors could not be relied on to provide strength during re-entry for fuselage bending, torsion, and aerodynamic pressure. The mid-fuselage had to accommodate Designing to Minimize Thus, the classical design approach the quantity, size, weight, location, Local Deflections for ascent was not possible for re-entry. stiffness, and limitations of known and The Orbiter skin was covered with more The bulkheads at each end of the unknown payloads. An innovative than 30,000 silica tiles to withstand the payload section and the longerons on design approach needed to provide a heat of re-entry. These tiles had a each side required additional strength. statically determinant attachment system limited capacity to accommodate To reduce weight and thermal distortion, between the payloads and mid-fuselage. structural deflections from thermal engineers designed the doors using This would decouple the bending, gradients. The European supersonic graphite epoxy. This was the largest twisting, and shear loads between the Concorde passenger aircraft (first flown composite structure on any aircraft or two structures, thus enabling engineers in 1969 and in service from 1976 to spacecraft at the time. to design both without knowing the stiffness characteristic of each. 2003) and the SR- 71 US military 272 Engineering Innovations aircraft encountered significant thermal protected the attached silica tile as as at several times during re-entry. gradients during flight. The design well as simplified the design and Engineers generated 120 thermal approach in each was to reduce stresses manufacture of the Orbiter airframe. math models for specific regions of induced by the thermal gradients by the Orbiter. Temperatures were NASA developed these design enabling expansion of selected regions extrapolated and interpolated to nodes criteria so that if the thermal stresses of the structure; e.g., corrugated within these thermal math models. reduced the mechanical stresses, the wing skins for the SR- 71 and “slots” reductions would not be considered in in the Concorde fuselage. After
Details
-
File Typepdf
-
Upload Time-
-
Content LanguagesEnglish
-
Upload UserAnonymous/Not logged-in
-
File Pages16 Page
-
File Size-