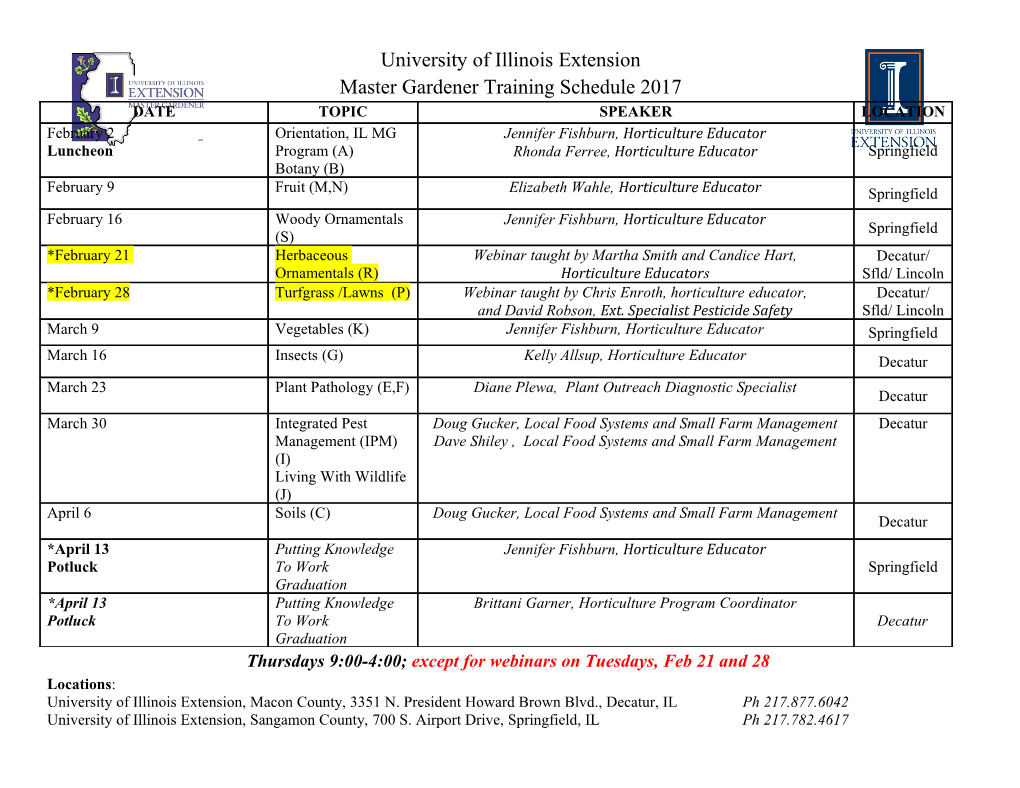
Metal Oxide Nanostructures and their Applications (ISBN 1-58883-170-1) Edited by Ahmad Umar, Najran University, Saudi Arabia Yoon-Bong Hahn, Chonbuk National University, South Korea. Published by American Scientific Publishers, CA, USA, (http://www.aspbs.com/mona/) October 2009 5-Volume Set, 4,000 pages, Hardcover Volume 4 Applications (Part-2) Chapter 13 Bioactive Bioceramic Coatings. Part II: Coatings on Metallic Biomaterials (Vol.4, pp 479-509) Proof-reading Corrections: No. Original Correction 1. Reference [39] 39. A. K. Lynn and D. L. DuQuesnay, Biomaterials 23, 1947 (2002). 2. Reference [97] 97. M. Wei, A. J. Ruys, B. K. Milthorpe, C. C. Sorrell, and J. H. Evans, J. Sol–Gel Sci. Techn. 21, 39 (2001). 3. Figure 6b ⊕ PO ∇ CO Titania 4 3 48 h ⊕ ∇ ⊕ ⊕ ∇∇ ⊕ ⊕ ⊕ ⊕ ⊕ ∇ 24 h ∇ ⊕⊕ Reflectance /arb. units /arb. Reflectance 0 h 2000 1600 1200 800 400 Wavelength /cm-1 CHAPTER 13 Bioactive Bioceramic Coatings: Part II. Coatings on Metallic Biomaterials Jin-Ming Wu1,Min Wang 2 1Department of Materials Science and Engineering, Zhejiang University, Hangzhou 310027, P. R. China 2Department of Mechanical Engineering, The University of Hong Kong, Pokfulam Road, Hong Kong CONTENTS 1. Introduction ..................................... 2 2. Bioactive Bioceramic Coatings ....................... 2 2.1. Physical Deposition ........................... 2 2.2. Sol–Gel Process .............................. 5 2.3. Electrocrystallization, Electrophoretic Deposition, and Electrostatic Spray Deposition ................ 6 2.4. Biomimetic Process ........................... 7 2.5. Other Coating Techniques ..................... 10 3. Nanostructured Titania for In Vitro Apatite Deposition .... 10 3.1. Coating Approaches ......................... 11 3.2. Chemical Modification Approaches .............. 14 4. Composite Coatings .............................. 20 4.1. Ceramic/Metal Composite Coatings ............. 20 4.2. Ceramic/Ceramic Composite Coatings ........... 21 4.3. Ceramic/Polymer Composite Coatings ........... 22 5. Bioceramic Coatings Incorporated with Biomolecules ..... 24 6. Concluding Remarks ............................. 25 References ..................................... 25 ISBN: 1-58883-170-1 Metal Oxide Nanostructures and Their Applications Copyright © 2010 by American Scientific Publishers Edited by Ahmad Umar and Yoon-Bong Hahn All rights of reproduction in any form reserved. Volume 4: Pages 1–31 1 2 Bioactive Bioceramic Coatings: Part II. Coatings on Metallic Biomaterials 1. INTRODUCTION The concept of bioactive bonding, which is defined as the direct chemical bonding of biomaterials to the surrounding tissue through the formation of fine hydroxylap- atite nanocrystals at the tissue-implant interface, opened a new era of design, manufac- ture, and application of biomaterials, when Hench et al. published their epoch-making paper in 1971 [1]. Although various bioactive glasses, ceramics, and glass-ceramics have been developed since the 1970s, most of these so-called bioactive bioceramics are not suitable for the load-bearing conditions for the replacement of bone or teeth, due to their poor mechanical properties. Compared to bioceramics and biomedical polymers, metals used in the medical field possess high mechanical properties, long fatigue life, good wear resistance, and excellent formability. Some of these metals exhibit additional properties of good corrosion resistance and acceptable biocompatibility and hence are good candidates for implants for high load-bearing applications. Metallic biomateri- als, such as commercially available pure Ti (CPTi), Ta, Nb, 316L stainless steel, Co–Cr, Co–Cr–Mo, and Co–Ni–Cr alloys, have been widely used in restorative surgery [2, 3]. However, none of the metallic biomaterials is bioactive. When they are implanted in the body, a fibrous capsule is formed to separate them from the host tissue [4]. There- fore, numerous efforts have been made to introduce a bioactive surface on the metallic biomaterials so that implants of these surface-modified metals can be bounded to the surrounding tissue shortly after implantation. In the first chapter of this two-chapter series on bioactive bioceramic coatings, we reviewed the concept of bioactivity, in vivo and in vitro evaluation methods for bioactivity, mechanisms of apatite formation on biomaterials, and bioactive bioceramic coatings on non-metallic biomaterials. This chapter concentrates on various approaches used to intro- duce a bioactive surface on metallic biomaterials, with special attentions paid to the bone- bonding ability of nanostructured titania thin films. For most systems, another major effect of coating metals with a bioceramic is the improvement in corrosion resistance and wear resistance of metal implants [5–7]. 2. BIOACTIVE BIOCERAMIC COATINGS Hench et al. in 1970 found that the Na2O–CaO–SiO2–P3O5 system glasses of certain com- positions formed a direct chemical bonding to the surrounded bone tissue without being separated by a fibrous tissue and hence started the development of bioactive glasses [8]. In the following decades, bioactive ceramics, such as hydroxylapatite (HA) and trical- cium phosphate (TCP), and bioactive glass-ceramics, such as Ceravital@ and A–W glass- ceramics, were invented [9]. Perhaps with the exception of A–W glass-ceramics, bioactive glasses, ceramics or glass-ceramics themselves are not strong enough to be used for load- bearing applications. However, these materials can be deposited on the surface of strong, stiff, and tough metallic substrates in order for the metals to have bioactivity, which pro- vides a solution to the clinical needs for strong and bioactive implants. The mid-1980s witnessed rapid industrial development of coating orthopedic and dental prostheses with HA using the plasma spraying technique, which has become an industrial gold standard. Since then, various techniques have been investigated to coat bioactive ceramics, mostly HA, on metallic substrates. 2.1. Physical Deposition 2.1.1. Thermal Spraying Plasma spraying [10–24] is the most widely used coating technique to form bioactive bio- ceramic coatings on metallic biomaterials. A typical plasma spraying operation employs plasma, or ionized gas, to partially melt and carry the ceramic particulates onto the surface of the substrate. During the coating process, the substrate is maintained at a rel- atively low temperature (generally lower than 300C) so that the mechanical properties of the metallic implants will not be compromised. HA coatings with a typical thickness Bioactive Bioceramic Coatings: Part II. Coatings on Metallic Biomaterials 3 of 40∼60 m can be obtained. HA feed stock powders are generally synthesized using different chemical reactions [25, 26]. However, bovine- or human bone-derived HA has also been used [22, 23]. Using flame-spheroidized HA powders as feedstock was found to decrease coating porosity and increase deposition efficiency [18]. In vivo evaluation confirmed that plasma-sprayed HA coatings led to an earlier and better physiological integration of the implant with the bone [21, 27, 28]. However, it was reported that plasma-sprayed HA coatings on titanium did not contribute to the bone- implant interfacial strength at 12 weeks after implantation and one year after loading, although the bone apposition ability improved significantly as compared to the plasma- sprayed titanium coating and uncoated titanium implants [29]. The temperature generated in the plasma exceeds 10,000C, which makes it difficult to control the composition, crystal structure, and crystallinity of the resultant coating. HA coatings produced by the plasma spraying often contain considerable amounts of amorphous calcium phosphate (ACP) and small amounts of crystalline phases other than HA, such as - and -TCP [30] and tetracalcium phosphate (TTCP) [31]. At the coating/titanium interface deposited apatite may react with titanium dioxide that resulted from oxidation of titanium with plasma gas, or it may be thermally decom- posed due to catalysis of titanium [32]. This adds to the complexity of controlling the plasma-spraying of HA coatings. The interfacial strength between the HA coating and the substrate is also insufficient due to the existence of micro-cracks as a result of the high residual stress generated due to the large difference between the linear thermal expansion coefficients (CTE) of ceram- ics (14.5 × 10−6 K−1 for HA [33]) and the metallic substrates (10.1 × 10−6 K−1 for CPTi [33]), especially for thick HA coatings [34–36]. A study on the HA coating on Ti-6Al-4V substrates revealed clearly a decreased bonding strength with increasing residual stress [35, 36]. In bonding tests, the fracture occurred mainly inside the HA coating under a low residual stress. Under a high stress, the fracture tended to occur along the coating- substrate interface. A plasma-sprayed HA coating with a thickness of 200 m exhibited higher residual stress than that having a 50 m thickness [35]. Introducing an interme- diate layer as a bond coat was suggested to effectively improve the adhesion strength between the HA coatings and the substrates [22, 23, 37, 38]. However, careful selection of the intermediate layer is important. It should match with both the top coating and the substrate. Otherwise, an adverse effect will occur. The intermediate layers of plasma- sprayed titanium and zirconia were found to reduce the interfacial strength between the plasma-sprayed HA and CPTi substrates, due to the reduction in the contact area between the intermediate layers and the HA coatings
Details
-
File Typepdf
-
Upload Time-
-
Content LanguagesEnglish
-
Upload UserAnonymous/Not logged-in
-
File Pages32 Page
-
File Size-