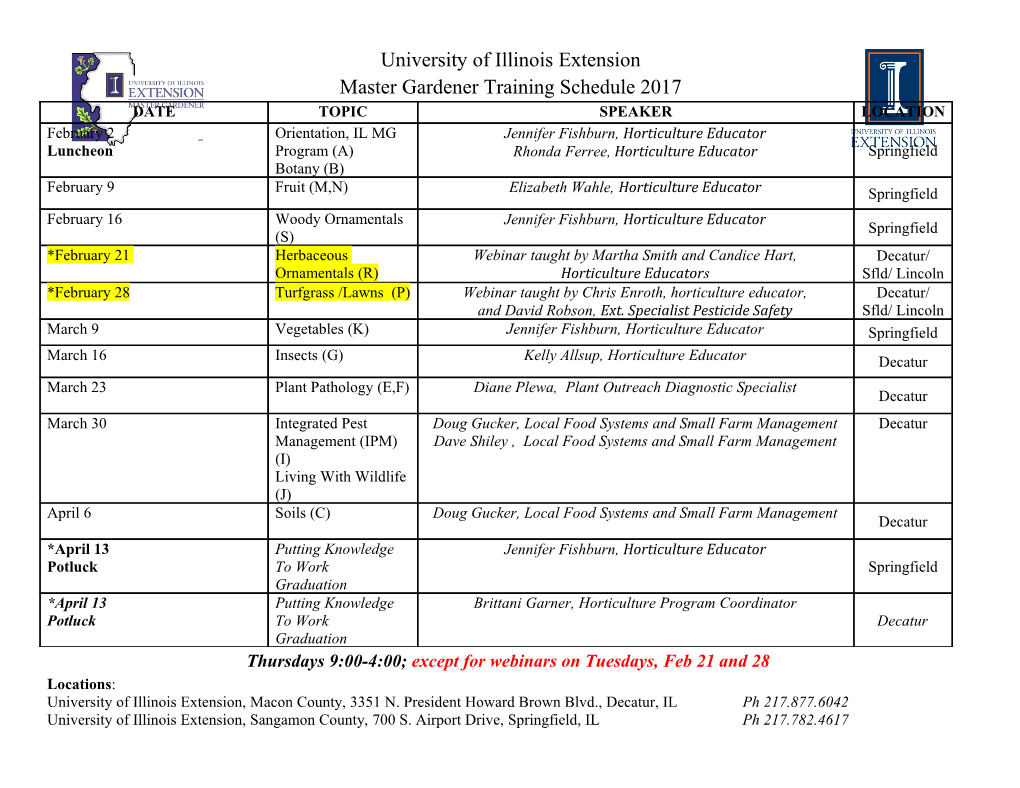
Discussion Paper | Discussion Paper | Discussion Paper | Discussion Paper | Atmos. Chem. Phys. Discuss., 14, 28685–28755, 2014 www.atmos-chem-phys-discuss.net/14/28685/2014/ doi:10.5194/acpd-14-28685-2014 © Author(s) 2014. CC Attribution 3.0 License. This discussion paper is/has been under review for the journal Atmospheric Chemistry and Physics (ACP). Please refer to the corresponding final paper in ACP if available. Interactions of bromine, chlorine, and iodine photochemistry during ozone depletions in Barrow, Alaska C. R. Thompson1,*, P. B. Shepson1,2, J. Liao3,**, L. G. Huey3, E. C. Apel4, C. A. Cantrell4, F. Flocke4, J. Orlando4, A. Fried4,*, S. R. Hall4, R. S. Hornbrook4, D. J. Knapp4, R. L. Mauldin III4, D. D. Montzka4, B. C. Sive5,***, K. Ullmann4, P. Weibring4, and A. Weinheimer4 1Department of Chemistry, Purdue University, West Lafayette, Indiana, USA 2Department of Earth, Atmospheric, and Planetary Sciences and Purdue Climate Change Research Center, Purdue University, West Lafayette, Indiana, USA 3School of Earth and Atmospheric Sciences, Georgia Institute of Technology, Atlanta, Georgia, USA 4National Center for Atmospheric Research, Boulder, Colorado, USA 5Institute for the Study of Earth, Oceans, and Space, University of New Hampshire, Durham, New Hampshire, USA *now at: Institute of Arctic and Alpine Research, University of Colorado Boulder, Boulder, Colorado, USA 28685 Discussion Paper | Discussion Paper | Discussion Paper | Discussion Paper | **now at: Chemical Sciences Division, Earth System Research Laboratory, NOAA, Boulder, Colorado, USA and Cooperative Institute for Research in Environmental Sciences (CIRES), University of Colorado-Boulder, Colorado, USA ***now at: National Park Service, Lakewood, Colorado, USA Received: 30 July 2014 – Accepted: 22 October 2014 – Published: 19 November 2014 Correspondence to: C. R. Thompson ([email protected]) Published by Copernicus Publications on behalf of the European Geosciences Union. 28686 Discussion Paper | Discussion Paper | Discussion Paper | Discussion Paper | Abstract The springtime depletion of tropospheric ozone in the Arctic is known to be caused by active halogen photochemistry resulting from halogen atom precursors emitted from snow, ice, or aerosol surfaces. The role of bromine in driving ozone depletion events 5 (ODEs) has been generally accepted, but much less is known about the role of chlo- rine radicals in ozone depletion chemistry. While the potential impact of iodine in the High Arctic is more uncertain, there have been indications of active iodine chemistry through observed enhancements in filterable iodide, probable detection of tropospheric IO, and recently, detection of atmospheric I2. Despite decades of research, significant 10 uncertainty remains regarding the chemical mechanisms associated with the bromine- catalyzed depletion of ozone, as well as the complex interactions that occur in the polar boundary layer due to halogen chemistry. To investigate this, we developed a zero- dimensional photochemical model, constrained with measurements from the 2009 OA- SIS field campaign in Barrow, Alaska. We simulated a 7 day period during late March 15 that included a full ozone depletion event lasting 3 days and subsequent ozone re- covery to study the interactions of halogen radicals under these different conditions. In addition, the effects of iodine added to our base model were investigated. While bromine atoms were primarily responsible for ODEs, chlorine and iodine were found to enhance the depletion rates and iodine was found to be more efficient per atom at 20 depleting ozone than Br. The interaction between chlorine and bromine is complex, as the presence of chlorine can increase the recycling and production of Br atoms, while also increasing reactive bromine sinks under certain conditions. Chlorine chemistry was also found to have significant impacts on both HO2 and RO2. The results of this work highlight the need for future studies on the production mechanisms of Br2 and 25 Cl2, as well as on the potential impact of iodine in the High Arctic. 28687 Discussion Paper | Discussion Paper | Discussion Paper | Discussion Paper | 1 Introduction The importance of halogen chemistry in Polar Regions has been well established over the past few decades since the observation of near-surface boundary layer ozone depletion in the 1980’s (Oltmans and Komhyr, 1986; Barrie et al., 1988; Bottenheim 5 et al., 1990). Since that time, ozone depletion events (ODEs) have been observed at numerous Arctic and Antarctic locations (e.g., Bottenheim et al., 2002; Saiz-Lopez et al., 2007b; Simpson et al., 2007; Oltmans et al., 2012). ODEs are characterized by episodic depletions of tropospheric ozone from background mole ratios of approxi- mately 35 parts per billion by volume (ppbv) to less than 2 ppbv over periods of hours 10 to days. These events are known to occur following the onset of polar sunrise, and continue through polar spring, when temperatures are low and snow and sea ice are still present, with a stable atmospheric boundary layer (Simpson et al., 2007). Photochemical reactions involving halogen radicals, notably bromine, are thought to be the primary cause of ODEs (see Simpson et al. (2007) for a review). The chemical 15 destruction of O3 by Br can be described by Reactions (R1)–(R3) (Platt and Hönninger, 2003). Br2 + hν → 2Br (R1) Br + O3 → BrO + O2 (R2) BrO + BrO → Br2 + O2 (R3) 20 The efficiency of Br atoms in destroying ozone is due primarily to its relative lack of atmospheric sinks, and thus its relatively high gas-phase concentration, as well as its ability to recycle and regenerate from temporary sink species. Bromine atoms do not react appreciably with methane or other saturated hydrocarbons; thus, its primary sinks (other than O3) consist of a few oxygenated volatile organic compounds (OVOCs) (e.g., 25 aldehydes) or unsaturated hydrocarbons, which result in production of HBr, and HOx species. BrO can react to regenerate a Br atom (via Reaction (R3), followed by R1), or react with HO2 to produce HOBr, which can in turn lead to the production of two 28688 Discussion Paper | Discussion Paper | Discussion Paper | Discussion Paper | Br atoms through a heterogeneous reaction mechanism termed the bromine explosion (Tang and McConnell, 1996; Vogt et al., 1996), as shown below. BrO + HO2 → HOBr + O2 (R4) HOBr(g) → HOBr(aq) (R5) − + 5 → HOBr(aq) + Br(aq) + H(aq) Br2(aq) + H2O (R6) Br2(aq) → Br2(g) (R7) The production of Br2 can thus be sustained on saline snow, ice, and aerosol sur- faces, as has been confirmed in laboratory studies that have observed production of Br2 and BrCl from aqueous and frozen halide surfaces exposed to HOBr (Fickert et al., 10 1999; Adams et al., 2002; Huff and Abbatt, 2002), as well as in a recent field-based study that observed Br2 production from sunlit snowpacks in Barrow, Alaska (Pratt et al., 2013). The presence of chlorine chemistry in the Arctic has been well recognized through in- direct measurements of hydrocarbons (Jobson et al., 1994; Ariya et al., 1998; Keil and 15 Shepson, 2006; Tackett et al., 2007) and through detection of photolyzable chlorine species (defined as [Cl2 + HOCl]) (Impey et al., 1997); however, few direct measure- ments of chlorine species have been reported. The only currently reported measure- ments of ClO were by Tuckermann et al. (1997), who detected ClO at Spitsbergen. Un- like bromine, chlorine radicals efficiently oxidize a wide-range of pollutants and volatile 20 organic compounds (VOCs), often with faster rate coefficients than analogous reactions by the hydroxyl radical (OH); thus, chlorine has an abundance of atmospheric sinks. Estimates of polar region Cl atom concentrations using hydrocarbon decay methods are typically in the range of 104–105 molecules cm−3 (Jobson et al., 1994; Ariya et al., 1998; Boudries and Bottenheim, 2000), approximately 2–3 orders of magnitude lower 25 than analogous estimates of Br (Cavender et al., 2008). Like bromine, chlorine can react directly with O3, generating a ClO radical via Re- action (R8). The presence of ClO may also promote bromine-induced depletion of O3 through the fast cross-reaction of BrO and ClO that serves to regenerate Br atoms 28689 Discussion Paper | Discussion Paper | Discussion Paper | Discussion Paper | (Reaction R9) (Le Bras and Platt, 1995; Platt and Hönninger, 2003). Cl + O3 → ClO + O2 (R8) BrO + ClO → Br + OClO (or Cl + O2) (R9) Due to analytical challenges, few tropospheric observations of Cl2 and ClO exist, there- 5 fore the role of Cl in ozone depletion events remains uncertain and has been much de- bated. Typical estimated Cl concentrations are likely too low for chlorine to be a signif- icant direct contributor to ozone depletion. However, elevated levels of Cl2 (exceeding 100 pptv) were recently observed during the Ocean–Atmosphere–Sea Ice-Snowpack (OASIS) 2009 campaign in Barrow, Alaska using chemical ionization mass spectrom- 10 etry (CIMS) (Liao et al., 2014). The impact that such high levels of Cl2 could have on ODEs or on the oxidation chemistry of the Arctic troposphere has not been fully investi- gated. Although Reaction (R9) can act to enhance the rate of ozone depletion by prop- agating the Br cycle, formaldehyde, propanal, acetaldehyde and HO2 are all produced as by-products of VOC oxidation by Cl and are efficient sinks for Br or BrO radicals 15 (Shepson et al., 1996; Sumner et al., 2002). Therefore, it is likely that the interaction between chlorine and bromine is complex. Modeling studies simulating ozone deple- tion events often use BrCl as the primary source of Cl atoms (Calvert and Lindberg, 2003; Piot and Von Glasow, 2008), and thus [Cl] is quite low and often insignificant. Few have added significant Cl2 sources (Sander et al., 1997; Piot and von Glasow, 20 2009). As a result, our understanding of chlorine chemistry in the Arctic is limited. Iodine may play a significant role in the photochemical reactions leading to ODEs (Sander et al., 1997; Saiz-Lopez et al., 2007b, 2008; Mahajan et al., 2010) based on recent ground-based and satellite observations of IO of up to 20–50 pptv over snow and ice-covered surfaces in coastal Antarctica (Saiz-Lopez et al., 2007a, b; Schön- 25 hardt et al., 2008; Frieß et al., 2010).
Details
-
File Typepdf
-
Upload Time-
-
Content LanguagesEnglish
-
Upload UserAnonymous/Not logged-in
-
File Pages36 Page
-
File Size-