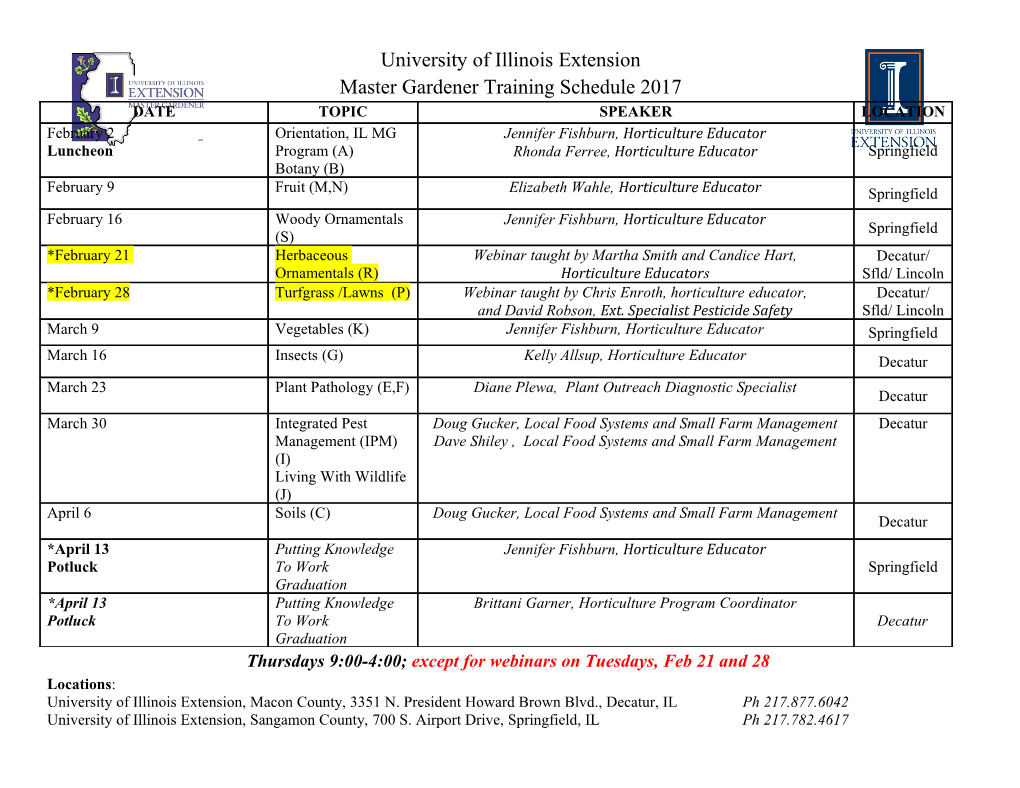
Journal of Hazardous Materials 183 (2010) 648–654 Contents lists available at ScienceDirect Journal of Hazardous Materials journal homepage: www.elsevier.com/locate/jhazmat 20 kHz sonoelectrochemical degradation of perchloroethylene in sodium sulfate aqueous media: Influence of the operational variables in batch mode Verónica Sáez a, María Deseada Esclapez b, Ignacio Tudela a, Pedro Bonete b, Olivier Louisnard c,d, José González-García a,∗ a Grupo de Nuevos Desarrollos Tecnológicos en Electroquímica: Sonoelectroquímica y Bioelectroquímica, Ap. Correos 99, 03080 Alicante, Spain b Grupo de Fotoquímica y Electroquímica de semiconductores, Departamento de Química Física e Instituto Universitario de Electroquímica, Universidad de Alicante, Ap. Correos 99, 03080 Alicante, Spain c Centre RAPSODEE, Ecole des Mines Albi, F-81013 Albi, France d Université de Toulouse, Mines Albi, CNRS, F-81013 Albi, France article info abstract Article history: A preliminary study of the 20 kHz sonoelectrochemical degradation of perchloroethylene in aqueous Received 12 May 2010 sodium sulfate has been carried out using controlled current density degradation sonoelectrolyses in Received in revised form 15 July 2010 batch mode. An important improvement in the viability of the sonochemical process is achieved when Accepted 16 July 2010 the electrochemistry is implemented, but the improvement of the electrochemical treatment is lower Available online 23 July 2010 when the 20 kHz ultrasound field is simultaneously used. A fractional conversion of 100% and degra- dation efficiency around 55% are obtained independently of the ultrasound power used. The current Keywords: efficiency is also enhanced compared to the electrochemical treatment and a higher speciation is also Perchloroethylene Sonoelectrochemistry detected; the main volatile compounds produced in the electrochemical and sonochemical treatment, Chlorinated compounds trichloroethylene and dichloroethylene, are not only totally degraded, but also at shorter times than in Dechlorination the sonochemical or electrochemical treatments. Groundwater © 2010 Elsevier B.V. All rights reserved. 1. Introduction binations of sonoelectrochemistry with other technologies, but also of the applications of sonoelectrochemistry for non-chemical Chlorinated compounds have been revealed as a strong recalci- treatments [11] or for the mediated synthesis of disinfectant trant group of pollutants, found in all kinds of water sources [1].As agents, such as hypochlorite [12]. However, the most common a consequence of the poor efficiency of the biological methods for approach in the sonoelectrochemical treatment (SECT) is direct the degradation of chlorinated compounds, a large number of alter- sonoelectrolysis in the configuration named “electrode-apart- native technologies have emerged and been developed in the last transducer” [13]. With this kind of experimental set-up, Trabelsi decade. These include not only simple procedures such as catalytic et al. [14] analyzed the sonoelectro-oxidation of aqueous solu- hydrogenation [2], metal alloys in dilute aqueous alkaline solutions tions of phenol + 0.5 g L−1 NaCl, and compared the results with [3] or other techniques such as photochemistry [4], sonochemistry those obtained by sonochemical (SCT) and electrochemical (ECT) [5] and also electrochemistry [6], but also combined treatments treatments used separately. Both 20 and 540 kHz frequencies and such as reductive dehalogenation and biodegradation [7], which a constant current density of 6.8 mA cm−2 were used during the are being considered as possible methods for the degradation of sonoelectrolyses, with nickel foam as the cathode and platinized chlorinated organocompounds. titanium grid as the anode. Higher fractional conversions were Sonoelectrochemistry means the application of ultrasound obtained at both frequencies, but shorter times were needed at fields to electrochemical processes, and is an active research high frequency. The authors revealed that the process becomes line in many different applications [8]; specifically, in environ- unrealistic at low frequency due to the formation of toxic com- mental remediation we can find peer-reviewed papers since pounds such as quinones, but higher frequency did not yield the 1990s. Recently, there have been reports not only of com- toxic aromatic compounds, with only acetic and chloroacrylic bined multi-techniques for the destruction of organic pollutants acids detected. Better effects in the combined technology, sono- in water [9,10], which show the wide range of possible com- electrochemistry, were detected in contrast with the sonolytical and electrochemical route. The sonoelectro-oxidation of pollutants has been further used in the degradation of dye effluents such ∗ Corresponding author. Tel.: +34 965903855. as Sandolan Yellow [15], Procion Blue [16], and Meldova Blue E-mail address: [email protected] (J. González-García). [17], of pesticides such as 2,4-dihydroxybenzoic acid [18], 2,4- 0304-3894/$ – see front matter © 2010 Elsevier B.V. All rights reserved. doi:10.1016/j.jhazmat.2010.07.074 V. Sáez et al. / Journal of Hazardous Materials 183 (2010) 648–654 649 dichlorophenol [19], and of additives such as thiosulfate [20] and used for the sonochemical treatment of PCE [29] and charac- cyanides [21]. terized [30,31], which has been adapted for sonoelectrochemical Sonoelectroreduction is the other route, which has been used trials in electrode-apart-transducer configuration. Lead (4.5 cm × for environmental applications. The recovery of silver from photo- 2.5 cm × 0.3 cm) and lead dioxide (4 cm × 2.7 cm × 0.6 cm) plates graphic processing solutions [22], and the degradation of pollutants were used as cathodes and anodes, respectively. Lead was cho- present in water, such as chlorinated organocompounds [23,24] are sen as the working electrode for this approach because of its high examples of processes studied. Perchloroethylene (PCE) is a widely overpotential for the reduction of water [32], in spite of the theoret- used solvent in many areas of industry and has been reported as ical drawbacks from an environmental point of view. No erosion of a major intermediate in the degradation of other chlorinated com- this material was observed during the sonoelectrochemical exper- pounds. In our laboratory we are analyzing the degradation of this iments. The volume of the working solution was 200 mL. The gas compound using different approaches: (i) by electrochemical treat- phase over the solution presented a volume of 130 mL. A Micro pH ments with not only fundamental [25,26] but also applied [27,28] 2000 Crison pHmeter and a Crison conductimeter model 525 were studies; (ii) sonochemical treatments [29] and now (iii) sonoelec- used to measure the initial and final pH and conductivity of the trochemical treatments, as in the present study. solution. The pH and conductivity changed from the initial values (6.0 and 7.3 mS cm−1) to the final values (3.4 and 8.6 mS cm−1). 2. Experimental section 2.3. Analysis 2.1. Materials For the aqueous phase, analyses were carried out by GC/MS (HP Technologies 6890N), GC/ECD (Varian GC 3800 microECD), GC/FID Perchloroethylene, C2Cl4, 99.9% (Aldrich) was used without any (HP 5890 Serie II), HPLC-UV (Agilent 1100 HPLC-UV) and ionic chro- further purification. Aqueous solutions were prepared with puri- matography, IC, (690 Ion Chromatograph, Metrohm). Compounds fied water obtained from a Milli-Q UV Plus system (18.2 M cm in the gaseous phase were analyzed by GC/FID and GC/TCD. Detailed resistivity). Na2SO4 was dissolved to give a concentration of 0.05 M, information about the temperature programmes used can be found with the solutions subsequently purged with Argon. PCE was then in previous papers of the authors [27,28]. Due to the fact that the added and the solution stirred in a closed volumetric flask with pH was also higher than 3.5 during the sonoelectrolyses, chlorine a glass-covered magnetic bar. The solutions were left overnight evolution was disregarded. with slow stirring to dissolve and equilibrate. Care was taken to minimize the effects of evaporation losses by using nearly full vol- 3. Results and discussion umetric flasks with tight-fitting ground-glass stoppers that were further sealed with Teflon tape. The initial concentration of PCE Even using a specific sonoelectrochemical reactor for which was in the range of 60–452 M. geometry aspects are already established, in sonoelectrolysis a large number of operational variables have to be fixed. So, in addi- 2.2. Experimental set-up tion to the chemical operational variables, such as concentration of the starting material, solvent, pH, conductivity, convection condi- Bulk sonoelectrolyses were carried out in galvanostatic mode tions and temperature, and the pure electrochemical operational using a Thandar precision DC power supply. Fig. 1 shows the undi- variables, such as electrode materials, current density or electrode vided sonoelectrochemical reactor at the laboratory scale used. This potential, we also have to establish the specific ultrasound opera- experimental set-up is the 20 kHz sonochemical reactor previously tional variables, such as working frequency and ultrasound power. Therefore, an extensive study of a sonoelectrochemical process should imply a huge number of experimental trials, which is beyond the scope of this paper. In this study, we will analyze the combina- tion of a high power 20 kHz ultrasound field with the conditions previously reported for the electrochemical degradation of PCE electrolysis [27], and the influence of the ultrasound power as an operational variable. Two different trials were conducted: (i) sono- electrolyses in which samples were taken at different times along the process, and (ii) sonoelectrolyses in which samples were only taken at the beginning and at the end of the process. No differences in the results were detected in these two methods. We have carried out PCE sonoelectrolysis experiments at differ- ent ultrasound intensities of 1.84, 3.39, 5.09, 6.36 and 7.64 W cm−2 (i. e. 0.065, 0.120, 0.180, 0.225 and 0.270 W cm−3), with T =20◦C, 60 ppm (362 M), and the value of the working current density, jw, fixed at 3.5 mA cm−2.
Details
-
File Typepdf
-
Upload Time-
-
Content LanguagesEnglish
-
Upload UserAnonymous/Not logged-in
-
File Pages7 Page
-
File Size-