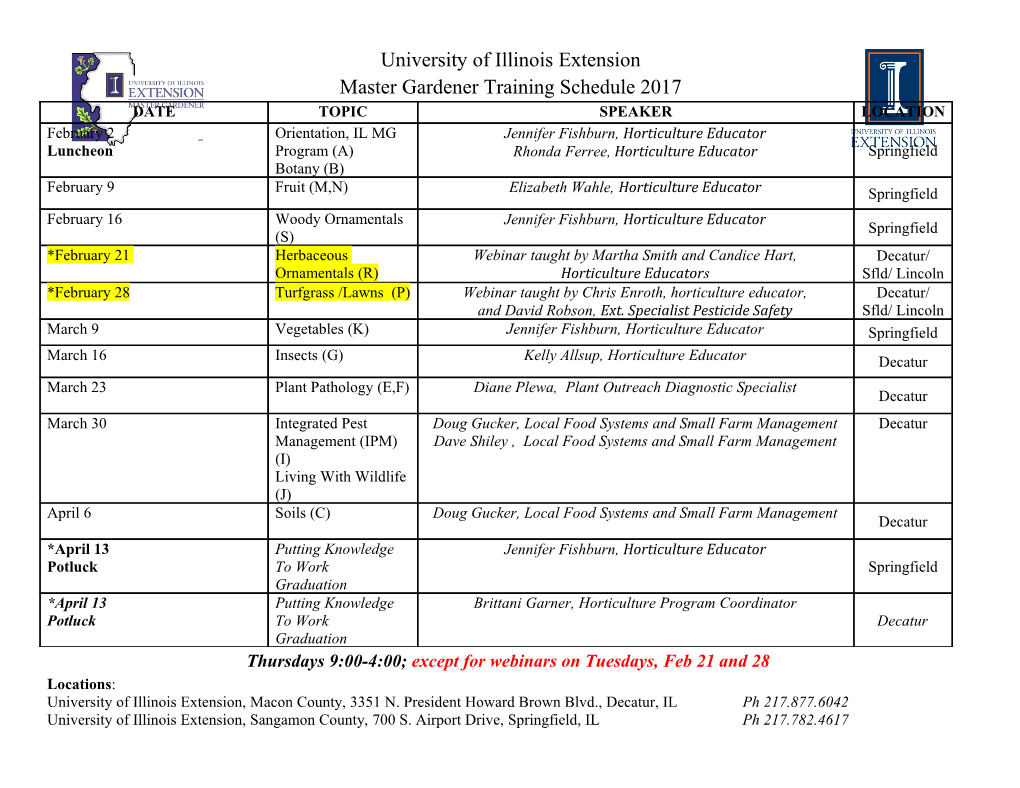
Annals of Discrete Mathematics 9 (1980) 291-296 © North-Holland Publishing Company ON CUTTING PLANES* A. SCHRIJVER Department of Mathematics, Eindhoven University of Technology, P.O. Box 513, Eindhoven, The Netherlands, and Mathematical Centre, Kruislaan 413, Amsterdam, The Netherlands We give a geometrical description of Chvatal's version of Gomory's cutting plane method. Restricting ourselves to rational spaces, we prove that the derived geometrical objects are polyhedra again, and that the method also works for unbounded polyhedra. 1. Introduction For any polyhedron P let P1 denote the convex hull of the lattice points contained in P. 1 If H is the half-space {x I wx,;;;; d}, where w is a vector whose components are relatively prime integers and d is a rational number, then one easily sees that H1 ={x I wx:;;;; LdJ}. Geometrically, H1 arises by shifting H until its bounding hyperplane contains lattice points. So for half-spaces H there is an easy way to determine H1. Since for each half-space H, the inclusion Pc H implies that Pr c Hr, we know that (1) where the intersection ranges over all half-spaces H with Pc H. We denote this intersection by P'. Below we show that P' is a polyhedron again, and that p<•> = P1 for some natural number t. (As usual, p<0> = P, and p(r+ll = p(•l'.) This is the essence of Chvatal's [1] formalization of Gomory's [4, 5, 6] cutting plane method for solving integer linear programming problems (cf. Rosenberg [11]). Chvatal's original method applies to bounded polyhedra in real space. However, the fact that the method works for these polyhedra follows from its effectiveness for rational polyhedra (see (i) of Section 4 below). Clearly, we may restrict the range of the intersection (1) to supporting half­ spaces, i.e., to half-spaces whose bounding hyperplane supports P. We shall see * Research supported by the Netherlands organization for the Advancement of Pure Research (Z.W.0.). 1 P is a polyhedron if P = {x I Ax"'= b} for some matrix A and some vector b. When using expressions like Ax"'= b we implicitly assume compatibility of sizes of matrices and vectors. We work within rational spaces, rather than real ones. So any matrix and any vector is supposed to be rational. - A lattice point is an integral vector, i.e., a vector with integer components. wx denotes the standard inner product of vectors w and x. LdJ denotes the lower integer part of a rational number d. For the theory of polyhedra and cones we refer to Griinbaum [7], Rockafellar [10] and Stoer and Witzgall [13]. 291 292 A. Schrijver below that we may restrict the intersection to finitely many supporting half­ spaces, namely to those half-spaces corresponding to a so-called totally dual integral system of linear inequalities determining P (cf. (ii) of Section 2 below). Therefore, the use of this method to determine P1 depends on the capability to find these half-spaces. 2. Two preliminaries (i) For each polyhedron P the set Pr is a polyhedron again. This not surprising fact can be derived from Motzkin's [9] theorem that each polyhedron P can be decomposed as P = Q + C, where Q is a bounded polyhedron and C is a polyhedral convex cone. Motzkin's theorem implies also that there are half-spaces H 1, .•• ,H., L 1 , •.• ,L. such that Pr=L1 n---nL., PcH1 n-• -nH., and Lie Hi for i = 1, ... , s (so the bounding hyperplanes of Li and H; are parallel). (ii) A system of linear inequalities Ax:;;;; b is called totally dual integral if the linear programming minimum min{yb I y;;;,,O, yA = w} (2) is attained by an integral vector y, for each integral vector w for which the minimum exists. Hoffman [8] and Edmonds and Giles [2] showed that if Ax:;;;; b is totally dual integral and b is integral, then each face of the polyhedron P = {x I Ax<.:: b} contains lattice points (i.e., P = Pr), That is, if b is integral and the right-hand side of the linear programming duality equation max{wx I Ax:;;;; b} = min{yb I y ;;;,,Q, yA = w} (3) is achieved by an integral vector y for each integral vector w for which the minimum exists, then also the left-hand side is achieved by an integral vector x, for each such vector w. It follows from the results of Giles and Pulleyblank [3] that for each polyhedron P there exists a totally dual integral system Ax<.:: b such that A is integral and P={x I Ax:;;;;b}. (In [12] it is shown that there exists a unique minimal such system, provided that P has nonempty interior, that is, provided that P has full dimension.) Chvatal's method to determine P1 as described in the present paper calls for algorithmic methods to determine such a totally dual integral system. 3. Theorems Theorem 1. For any polyhedron P the set P' is a polyhedron again. Proof. Let P = {x I Ax<.:: b }. We may suppose that A is an integral matrix, and, by (ii) of Section 2, that the system Ax:;;;; b is totally dual integral. We show that P' = {x I Ax.;;; Lb J} (where Lb J arises from b by taking componentwise lower integer parts), which yields that P' is a polyhedron. On cutting planes 293 First, P'c{x I Ax:;;;; LbJ}, as each linear inequality in the system Ax::;;;;b gives a half-space H, while the corresponding inequality in Ax::;;;; Lb j contains Hr. Conversely, suppose H = {x I wx::;;;; d} is a half-space containing P as a subset. We may suppose that the components of w are relatively prime integers, so Hr={x I wx::;;;; LdJ}. Now d;;;,,max {wx I Ax::;;;; b}=min {yb I y;;;,,O, yA = w}. (4) As the system Ax::;;;; b is totally dual integral we know that the minimum in (4) is achieved by some integral vector y0 . Therefore LdJ;;;,, Ly0 bj ;;;,,y0 LbJ, which yields that wx::;;;; LdJ for all x with Ax::;;;; Lb J. This implies {x I Ax::;;;; Lb J} c Hr. So {x !Ax:;;;; LbJ}cP'. Theorem 2. For any polyhedron P there exists a number t such that p<•> = Pr. Proof. We prove the theorem by induction on the dimension of the space, and on the (affine) dimension of (the affine hull of) P. If both are zero the theorem is easy. If the dimension of P is less than the dimension of the space, then P is contained in some hyperplane K. If K contains no lattice points, then it is easy to see that P1 = P' = \3. If K contains lattice points, then there exists an affine transformation of the space which brings K to the subspace K 0 of vectors with last component zero, and which brings the set of lattice points onto the set of lattice points. Moreover, the image of P is again a polyhedron, say P0 • By induction we know that in the space K0 we have: (P0 f> = (P0 )i, for some natural number t. Since each half-space H 0 of K 0 can be extended to a half-space Hof the original space such that H n K0 = H 0 and Hr n K 0 = (H0 )r, it follows that also in the original space (P0 )'0 = (P0)r. Since the collection of half-spaces and the set of lattice points are invariant under the affine transformation we know that also p<r>=Pr. Now suppose the dimension of P is equal to the dimension of the space. Let H = {x I wx::;;;; d} be a half-space containing Pr as a subset, such that Pis contained in some half-space {x I wx::;;;; d'}. We prove that there is a number s such that p<•> c H. As Pr is the intersection of a finite number of such half-spaces H (cf. (i) of Section 2) the theorem follows. Suppose, to obtain a contradication, that for no s we have that p<•> is contained in H. Since P'c{x I wx::;;;;Ld'J}, there exists an integer d">d such that p<•>c {x I wx::;;;; d"} for all s;;;,, r, for some number r, but for no s we have p<•> c {x I wx::;;;; d" - l}. Let K be the hyperplane {x I wx = d"}. We prove below that if Q is a polyhedron contained in {x I wx::;;;;d'1, then Q'nKc(QnK)'. By induction on u this implies that o<u> n Kc (Q n K)M for all u. Since the dimension of p<•> n K is less than the dimension of P, and since P(r) n K does not contain lattice points, we know by the induction hypothesis that (P<•> n K)'"> = ~ for some u. 294 A. Schrijver Therefore, p(r+u)nK=~, which implies that p(r+u+llc{x I wx:;;;d"-1}, con­ tradicting our assumption. We prove that for any polyhedron Q with Q c {x I wx:;;; d"} one has Q' n Kc ( Q n K)' (and equality follows). It is sufficient to show that for each half-space H with Q n Kc H there is a half-space G such that Q c G and Gr n Kc Hr. Let H = {x I vx:;;;; e} be such a half-space, where the vector v consists of relatively prime integers. As H:::iQnK= Qn{x 1-wx:;;;-d"}, (5) there exist, by Farkas' lemma, ,\ ~ 0, and v', e' such that v = v' - ,\ w, e ~ e' - ,\d", and Q c {x I v' x:;;; e'}.
Details
-
File Typepdf
-
Upload Time-
-
Content LanguagesEnglish
-
Upload UserAnonymous/Not logged-in
-
File Pages6 Page
-
File Size-