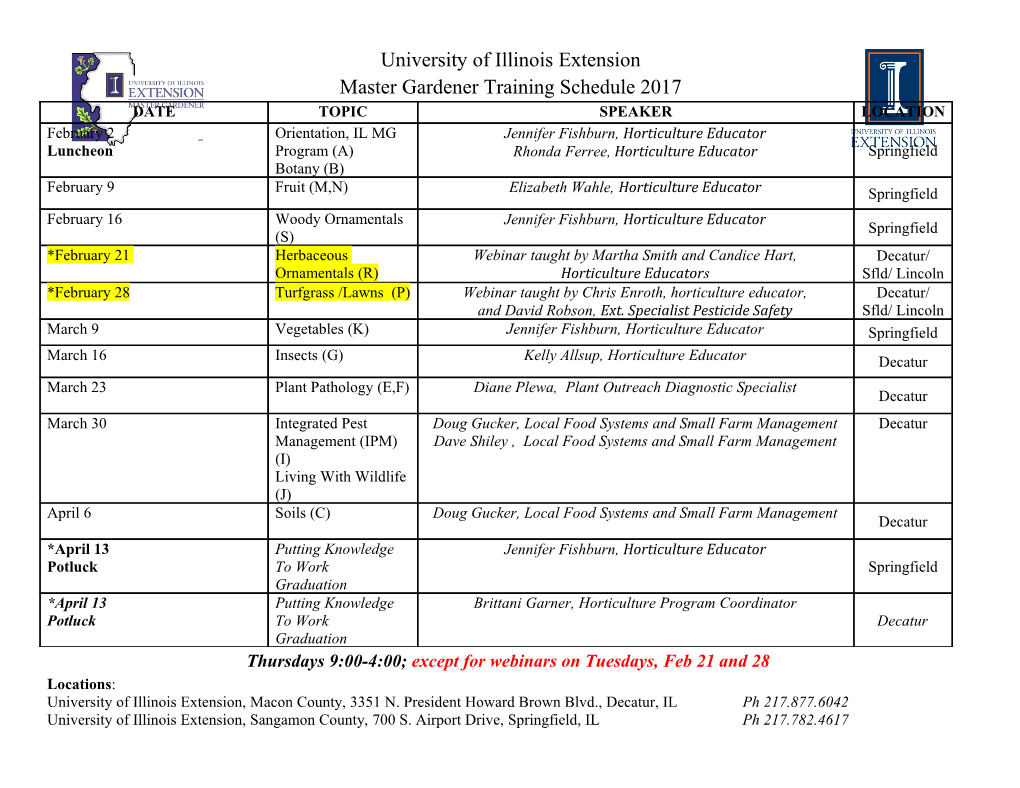
Earth and Planetary Science Letters 154Ž. 1998 221±235 Depleted spinel harzburgite xenoliths in Tertiary dykes from East Greenland: Restites from high degree melting Stefan Bernstein a,), Peter B. Kelemen b,1, C. Kent Brooks a,c,2 a Danish Lithosphere Centre, éster Voldgade 10, DK-1350 Copenhagen K, Denmark b Woods Hole Oceanographic Institution, Woods Hole, City, MA 02543, USA c Geological Institute, UniÕersity of Copenhagen, éster Voldgade 10, DK-1350 Copenhagen K, Denmark Received 28 April 1997; revised 19 September 1997; accepted 4 October 1997 Abstract A new collection of mantle xenoliths in Tertiary dykes from the Wiedemann Fjord area in Southeast Greenland shows that this part of the central Greenland craton is underlain by highly depleted peridotites. The samples are mostly spinel harzburgites with highly forsteritic olivinesŽ. Fo87± 94 , average Fo 92.7 . This, together with unusually high modal olivine contentsŽ. 70±)95% , places the Wiedemann harzburgites in a unique compositional field. Relative to depleted Kaapvaal harzburgites with comparable Fo in olivine, the Wiedemann samples have considerably lower bulk SiO2 Ž average 42.6 wt% versus 44±49 wt%. Spinel compositions are similar to those in other sub-cratonic harzburgites. Pyroxene equilibrium temperatures average 8508C, which is above an Archaean cratonic geotherm at an inferred pressure of 1±2 GPa, but low enough so that it is unlikely that the xenoliths represent residual peridotites created during Tertiary magmatism. Among mantle samples, the Wiedemann harzburgites are, in terms of their bulk composition, most similar to harzburgites from the ophiolites of Papua New GuineaŽ. PNG and New Caledonia Ž. NC . One hypothesis is that the Wiedemann harzburgites, along with PNG and NC harzburgites, formed via dissolution of pyroxene from previously depleted peridotites, possibly beneath a volcanic arc. If so, higher spinel CrrAl in Wiedemann samples may reflect a deeper origin compared to PNG and NC peridotites. Alternatively, using proposed primitive mantle compositions as a protolith, the Wiedemann harzburgites can be modeled as the residue after extraction of some 40% melt. The composition of this calculated hypothetical melt in terms of CaO, Al23 O , FeO, MgO and SiO 2 is similar to published experimental data on high degree melts of peridotite at 2±3 GPa. Munro-type komatiites lie close to these calculated and experimental melts but are slightly displaced towards low degree experimental melts at higher pressureŽ. e.g., 6 GPa . We conclude that the Wiedemann harzburgites formed as a residue after about 40% melting, and that they may represent shallow, refractory residues after polybaric melting initiated at pressures G7 GPa and continuing to relatively low pressuresŽ. 2±3 GPa or less . Extraction and aggregation of polybaric melts would produce liquids similar to Munro-type komatiites. q 1998 Elsevier Science B.V. Keywords: harzburgite; xenoliths; Greenland; partial melting; komatiite 1. Introduction Corresponding author. Tel.: q45 3814 2656. Fax: q45 3311 0878. E-mail: [email protected] Tel.: 508 457 2000, ext. 2956. E-mail: [email protected] The highly refractory nature of mantle xenoliths Tel.: 45 3532 2417. E-mail: [email protected] from beneath the Archaean Kaapvaal craton, South 0012-821Xr98r$19.00 q 1998 Elsevier Science B.V. All rights reserved. PII S0012-821XŽ. 97 00175-1 222 S. Bernstein et al.rEarth and Planetary Science Letters 154() 1998 221±235 Africa, was realized in the early 1970sŽ e.g.wx 1. 2. Occurrence and nature of the Wiedemann Fjord Since then, several authors have demonstrated that xenoliths mantle xenoliths from Archaean cratons, compared to those from Proterozoic and Phanerozoic crustal Mantle peridotite xenoliths in East Greenland were terrains, in general have higher modal olivine, found in erratic blocks by Brooks and Rucklidgewx 9 , forsterite contentŽŽ FosMgasatomic Mgr Mgq and their refractory nature was established by the ) r q Fe..)100 in olivine Ž often Fo91 . , and Cr ŽCr olivine-rich modal composition and highly forsteritic wx Al. in spinels 2±4 . These characteristics have been olivineŽ. Fo92.5 . Wiedemann Fjord was visited again explained in terms of extensive melt extraction from in the summer of 1995 and two main localities of the mantle, leaving a residue highly depleted in xenolith-bearing dykes were discoveredŽ. Fig. 1 . The basaltic componentsŽ e.g.wx 2,3,5±7. Further, be- dykes intrude Tertiary plateau basaltswx 10 , which cause of the high degrees of melting inferred, the were extruded between 58 and 56 Mawx 11,12 and refractory mantle material has been linked to the reach a thickness in excess of 6 kmwx 13 . The main extraction of komatiites in the Archaeanwx 8 . Here we basaltic volcanism terminated at about 47 Mawx 14 . present the mineral chemistry of a new suite of To the southwest, the basalts rest on gneissic base- spinel peridotite xenoliths of mantle origin that may ment with model ages between 3.02 and 2.79 Ga help to shed light on the connection between de- Ž.Sm±Nd and between 3.07 and 2.51 Ga Ž. Pb±Pb pleted lithospheric mantle and komatiites. wx15 . Further to the northeast, the area was affected Fig. 1. The xenolith-bearing dykes with the two main xenolith localitiesŽ. NS1 and 2 and NS4 . The dykes run N±S and are vertical Ž thick black lines. Dark graysbasalts of the Geikie Plateau Formation basalts. Stippled body is the 49 my ultramafic±mafic Lilloise intrusion. Insert gives the location of the larger map. S. Bernstein et al.rEarth and Planetary Science Letters 154() 1998 221±235 223 by Caledonian thrusts and granitic intrusionswx 16 . thopyroxene and chrome-spinel, interpreted as man- The basement is not exposed around Wiedemann tle xenocrystswx 9 . The mesostasis in the dykes con- Fjord, and it is unknown whether it is Archaean or sists largely of plagioclase and alkali feldspar. Small Caledonian. titanomagnetite grains are distributed throughout the The xenolith-bearing dykes are 40.48"0.27 Ma rock. The whole rock chemistry is that of an alkali Ž.Ar±Ar method; R. Duncan, pers. commun. , post- basaltŽ. Table 1 . dating both continental break-up, by roughly 15 Ma, Xenoliths occur in a central plane parallel to dyke and the last important magmatic episode, by 9±6 margins. The xenoliths have crudely discoidal shapes, Ma. The dyke location and their N±S strike and with large aspect ratios. They range in size from 2±3 vertical dip correlate with a N±S zone of prominent mm to 6 cm in maximum dimension, with an aver- faultingwx 13Ž. Fig. 1 . age of about 1±2 cm. In general, they are fresh but The xenolith-bearing dykes are 0.2±1.5 m wide in 2±3%, all olivine and orthopyroxene is replaced and occur in clusters of less than 5. They commonly by serpentine. About 50% of the xenoliths show 1±2 have a distinct yellowish alteration haloŽ 2±15 cm mm alteration rims where again olivine and orthopy- wide. , consisting of calcite and clay minerals. The roxene are replaced. The rest show little hydrother- dykes themselves weather to a dark brown colour. mal alteration. Invasion of melt from the host is only Subhedral, kaersutitic amphibole makes up about observed in few xenoliths. In some xenoliths, or- 50% of the matrix of the rock, in addition to 10% thopyroxene have reaction rimsŽ 1±2 mm wide, euhedral, microphenocrysts of clinopyroxene, which fine-grained. against the host dyke. Partial melting of is zoned from colourless cores to deep lilac-coloured the xenoliths, probably during heating and decom- rims. Other rare, large crystals include olivine, or- pression during ascent in the dykes, is indicated by Table 1 Compositions of average Wiedemann harzburgite; estimated primitive mantle; computed liquid in equilibrium with average Wiedemann harzburgite; experimental melts, their starting material; and the matrix of the nodule-bearing dyke ) SiO2223 TiO Al O FeO MnO MgO CaO Na 2 O Sum Mga Ol±Fo Average Wiedemann harzburgite 42.63 0.01 0.82 6.65 0.19 48.43 0.19 0.03 98.95 HZPUM primitive mantle 45.96 0.18 4.06 7.54 0.13 37.78 3.21 0.33 99.19 Pyrolite primitive mantle 45.10 0.20 3.30 8.00 0.15 38.10 3.10 0.04 97.99 38% melt of HZPUMŽ. computed liquid 51.39 0.46 9.34 9.00 0.03 20.40 8.15 0.86 99.63 81.78 93.54 40% melt of pyroliteŽ. computed liquid 48.81 0.49 7.02 10.03 0.01 22.60 7.47 0.96 97.39 81.69 93.50 P() kbar Kettle River start material 44.90 0.15 4.26 8.02 0.13 37.30 3.45 0.22 98.43 36% melting 30 48.98 0.48 11.06 9.45 19.71 8.78 0.77 99.23 80.50 93.02 52% melting 30 47.96 0.39 9.50 9.19 23.89 7.70 0.52 99.15 83.73 94.32 11% melting 60 44.97 1.01 6.37 12.64 23.26 9.05 0.80 98.10 78.46 92.16 HW start material 45.20 0.71 3.54 8.47 0.14 37.50 3.08 0.57 99.21 20 50.18 1.74 8.61 9.34 0.90 19.96 7.40 1.40 99.53 79.20 92.47 20 49.16 1.40 6.83 9.19 0.15 24.96 6.11 1.13 98.93 82.87 93.98 30 47.79 1.81 8.55 9.55 21.39 7.60 1.52 98.21 79.96 92.79 TQ start material 44.95 0.08 3.22 7.60 0.14 40.03 2.99 0.18 99.19 20 49.21 0.25 9.58 8.61 21.40 9.04 0.57 98.66 81.58 93.46 20 49.28 0.36 9.94 9.08 20.57 9.54 0.47 99.24 80.14 92.87 30 48.46 0.22 8.62 8.88 23.85 8.13 0.51 98.67 82.71 93.92 Nodule-bearing dykeŽ.
Details
-
File Typepdf
-
Upload Time-
-
Content LanguagesEnglish
-
Upload UserAnonymous/Not logged-in
-
File Pages15 Page
-
File Size-