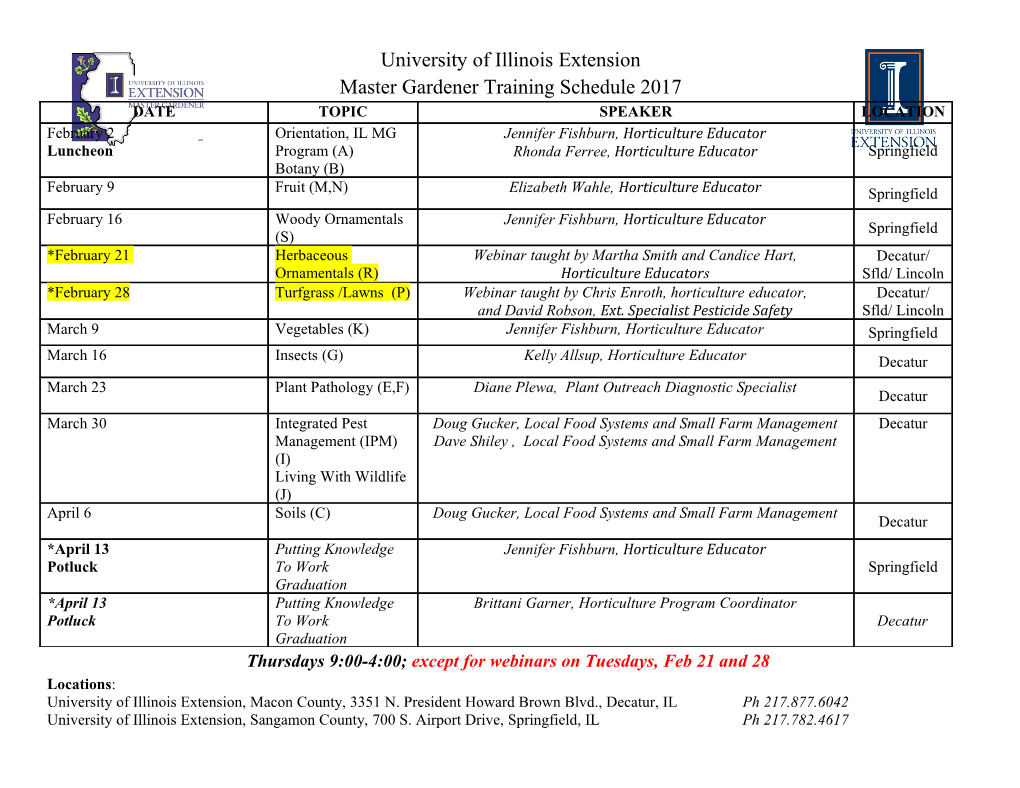
CERN Courier July/August 2016 Astrowatch C OMPILED BY M ARC TÜRLER , ISDC AND O BSERVATORY OF THE U NIVERSITY OF G ENEVA , AND CHIPP, U NIVERSITY OF Z URICH Neutron-star mergers create heaviest elements The origin of some of the heaviest chemical would result in stronger differences from one elements is due to rapid neutron capture, but galaxy to the other. the precise location where this cosmic alchemy Alexander Ji from the Massachusetts takes place has been under debate for several Institute of Technology, US, and colleagues decades. While core-collapse supernovae were lucky to fi nd extreme relative were thought to be the prime production site, a abundances of r-process elements in stars new study suggests that elements heavier than located in the ultra-faint dwarf galaxy zinc originate from the merger of two neutron Reticulum II. Although nearby and in orbit stars. Such a dramatic event would have been around the Milky Way, this galaxy was only responsible for the extreme heavy-element recently discovered and found to be among enrichment observed in several stars of an the most metal-poor galaxies known. This ancient dwarf galaxy called Reticulum II. means that Reticulum II formed all of its Nuclear fusion in the core of massive stars stars within about the fi rst three-billion Dana Berry/SkyworksDana Inc./The Digital, Foundation Kavli produces elements up to and including iron, years after the Big Bang, and is therefore which is a stable nucleus with the highest only enriched in elements heavier than binding energy per nucleon. Building heavier helium by a few generations of stars. nuclei requires energy to compensate for the Artist’s impression of two neutron stars High-resolution spectroscopic loss of nuclear binding and is therefore almost colliding – an event found to produce the measurements of the nine brightest stars impossible to achieve experimentally. But heaviest elements such as gold, lead and in Reticulum II carried out by the team under certain conditions, stars can produce uranium. indicate a very strong excess of europium heavier elements by allowing them to capture and barium compared with iron in seven protons or neutrons. For the r-process to work, nuclei need to of the stars. These abundances exceed by Neutron capture, which is unaffected by be under heavy neutron bombardment in two-to-three orders of magnitude those Coulomb repulsion, occurs either slowly conditions that are only found in dramatic in any other ultra-faint dwarf galaxy, (s) or rapidly (r). Slow neutron captures events such as a core-collapse supernova or in suggesting that a single rare event produced occur at a pace that allows the nucleus to mergers of two neutron stars. The supernova these r-process elements. The results also undergo beta decay prior to a new capture, hypothesis has long been the most probable show that this event could be a neutron-star and therefore to grow following the line of candidate for the r-process, whereas other merger, but not an ordinary core-collapse nuclear stability. The r-process, on the other scenarios involving rarer events, such as supernova. Although it is not possible to hand, causes a nucleus to accumulate many encounters between a neutron star and a black conclude that the majority of our gold and additional neutrons prior to radioactive hole, have only been considered since the uranium comes from neutron-star mergers, decay. The relative abundance of certain 1970s. One way to distinguish between the the study certainly gives more weight to You don’t have to count every atom elements therefore tells researchers whether two hypotheses is to study low-metallicity such a hypothesis in the 60-year-long debate nucleosynthesis followed an s- or an galaxies in which the enrichment of heavy about the origin of r-process elements. – we already have. r-process. The rare-earth element europium elements is low. This enables astrophysicists is a typical r-process element, as are gold, to determine if the enrichment is a continuous ● Further reading lead and uranium. process or the result of rare events, which A P Ji et al. 2016 Nature 531 610. Supplying science and industry around the world with specifically tailored properties and the purest copper products. Picture of the month For sputtering, cryogenic and vacuum applications requiring This beautiful image, showing an enormous bubble being blown into Cu 99.999 percent, RRR 1000 and above - or when every number counts. space by a young star, was chosen to celebrate the 26th anniversary of the Hubble Space Telescope. After so many stunning images captured by this exceptional satellite since its launch on 24 April 1990, it is diffi cult to surprise us. Like a soap bubble, the delicate Bubble Nebula is being blown into space by a super-hot star 45 times more massive that our Sun (located slightly off-centre on the upper-left side of the bubble). The strong stellar wind racing out at a speed of 6.4 million km/h sweeps up the cold interstellar gas in front of it, forming the outer edge of the bubble much like a snowplough piles up snow. Located in the constellation of Cassiopeia at a distance of NASA/ESA/Hubble (STScI/AURA) Heritage Team 7100 light-years, the nebula has a diameter of seven light-years – which is about one-and-a-half times the distance from our Sun to its nearest stellar neighbour, Alpha Centauri. 15 Ad.indd 1 04/05/2016 10:10 CERNCOURIER www. V OLUME 5 6 N UMBER 6 J ULY /A UGUST 2 0 1 6 CERN Courier July/August 2016 History Ghosts in the machine Los Alamos National Laboratory Christine Sutton describes the pioneering 1956 experiment that proved the existence of the neutrino, and how subsequent particle-beam experiments at CERN and elsewhere contributed to unearthing a further two neutrino types. In July 1956, in a brief paper published in Science, a small team based at the Los Alamos National Laboratory in the US presented results from an experiment at a new, powerful fi ssion reactor at the Savannah River Plant, in South Carolina. The work, they wrote, “verifies the neutrino hypothesis suggested by Pauli”. Clyde Cowan, Fred Reines, Kiko Harrison, Herald Kruse and Austin McGuire had demonstrated for the fi rst time that it was possible to detect neutrinos, setting in motion the new fi eld of neutrino phys- ics. The key ingredients were an intense source and a big detector, with more than a touch of ingenuity and patience. More than two decades previously, in 1930, Wolfgang Pauli had proposed that the “energy crisis” in nuclear beta decay – pre- sented by the continuous energy spectrum of the emitted electron – would be solved if the decaying nucleus also emitted a second, Fred Reines, left, and Clyde Cowan, at the controls of the undetected particle. This would allow the energy released to be Savannah River experiment, which discovered the electron shared between three objects, including the recoiling nucleus, and antineutrino in 1956. so yield electrons with a range of energies, just as observed. The new particle had to be neutral and have a relatively small mass. neutrinos produced during tests of atomic bombs to make a direct Pauli called his proposal “a desperate remedy”, in part because he detection of the elusive particle. He was soon joined in this strange thought that if such a particle did indeed exist, then it “would prob- pursuit by Clyde Cowan, a fellow researcher at Los Alamos, after ably have long ago been seen”. they were stranded together at Kansas Airport, where the conver- Nevertheless, Enrico Fermi took the possibility seriously and sation turned to the “supreme challenge” of detecting neutrinos. based his seminal work on beta decay, published in 1934, on a Reines had an idea to place a detector close to a bomb-test tower point-contact interaction in which a neutron decays to a proton, and use the timing of the detonation as a “gate” to minimise back- electron and (anti)neutrino: n → p e– ν–. Soon afterwards, Hans ground. But what kind of detector? He and Cowan decided on the Bethe and Rudolf Peierls calculated the cross-section for the recently developed medium of liquid scintillator, which could both inverse reaction in which a neutrino is absorbed, but when they act as a target for the inverse beta-decay reaction ν– p → e+ n, and found a value of about 10–44 cm2, the pair concluded that no one detect the emitted positrons via their annihilation to gamma rays. It would be able to detect neutrinos (Bethe and Peierls 1934). What was an audacious plan, not only in taking advantage of a bomb test they did not count on was the discovery of nuclear fi ssion – which but also in scaling up the use of liquid scintillator, which until then on a macroscopic scale produces copious numbers of neutrinos – or had been used only in quantities of about a litre. Reines and Cowan the ingenuity of experimentalists and, later, accelerator physicists. named it “Project Poltergeist”, to refl ect the neutrino’s ghostly nature. Notoriously, nuclear fi ssion was fi rst applied in the atomic bombs Remarkably, the Los Alamos director gave approval for the used towards the end of the Second World War. A few years later, experiment. However, in late 1952, Cowan and Reines were urged in 1951, Fred Reines, a physicist who had worked on the Manhat- to reconsider the more practical idea of using antineutrinos from a ▲ tan Project at Los Alamos, began to think about how to harness the nuclear reactor.
Details
-
File Typepdf
-
Upload Time-
-
Content LanguagesEnglish
-
Upload UserAnonymous/Not logged-in
-
File Pages2 Page
-
File Size-