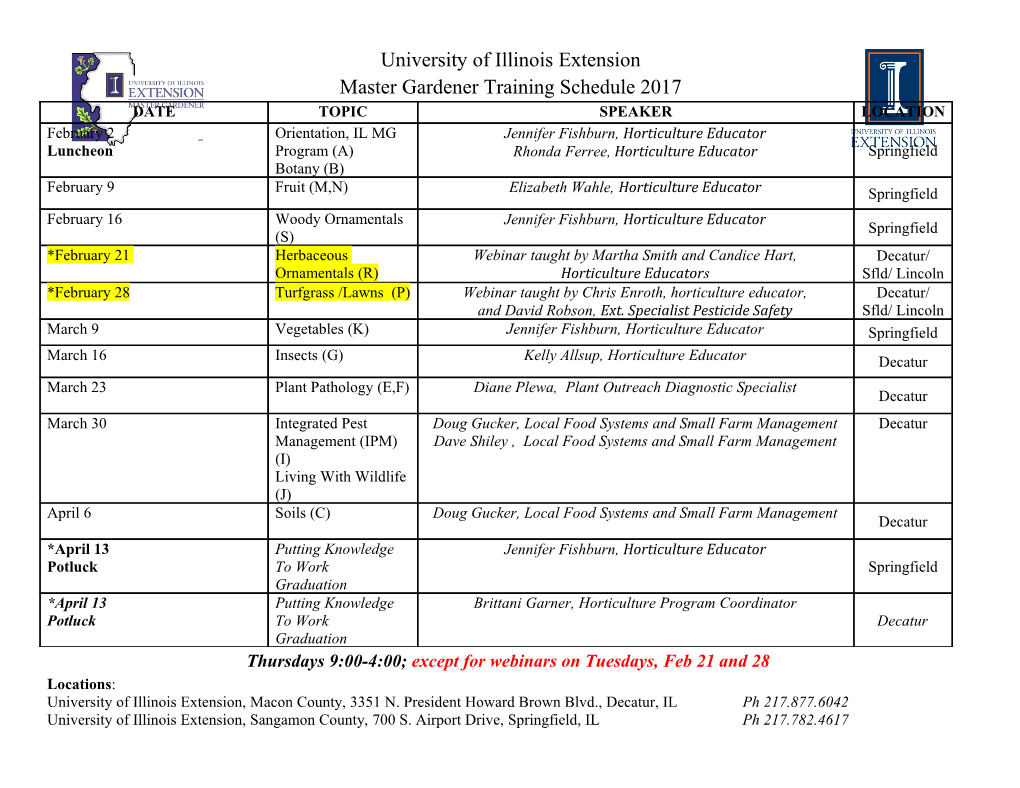
Downloaded from genesdev.cshlp.org on October 7, 2021 - Published by Cold Spring Harbor Laboratory Press ZCCHC8, the nuclear exosome targeting component, is mutated in familial pulmonary fibrosis and is required for telomerase RNA maturation Dustin L. Gable,1,2,3 Valeriya Gaysinskaya,2,3 Christine C. Atik,2,3 C. Conover Talbot Jr.,4 Byunghak Kang,5 Susan E. Stanley,1,2,3 Elizabeth W. Pugh,6 Nuria Amat-Codina,2,3 Kara M. Schenk,7 Murat O. Arcasoy,8 Cory Brayton,5 Liliana Florea,6 and Mary Armanios2,3,6,9,10 1Medical Scientist Training Program, Johns Hopkins University School of Medicine, Baltimore, Maryland 21205, USA; 2Department of Oncology, Johns Hopkins University School of Medicine, Baltimore, Maryland 21287, USA; 3Telomere Center, Johns Hopkins University School of Medicine, Baltimore, Maryland 21287, USA; 4Institute for Basic Biomedical Sciences, Johns Hopkins University School of Medicine, Baltimore, Maryland 21205, USA; 5Department of Comparative and Molecular Pathobiology, 6Department of Genetic Medicine, Johns Hopkins University School of Medicine, Baltimore, Maryland 21287, USA; 7Osler Medical Housestaff Training Program, Johns Hopkins University School of Medicine, Baltimore, Maryland 21205, USA; 8Department of Medicine, Duke University School of Medicine, Durham, North Carolina 27708, USA; 9Sidney Kimmel Comprehensive Cancer Center, Johns Hopkins University School of Medicine, Baltimore, Maryland 21287, USA Short telomere syndromes manifest as familial idiopathic pulmonary fibrosis; they are the most common premature aging disorders. We used genome-wide linkage to identify heterozygous loss of function of ZCCHC8, a zinc-knuckle containing protein, as a cause of autosomal dominant pulmonary fibrosis. ZCCHC8 associated with TR and was required for telomerase function. In ZCCHC8 knockout cells and in mutation carriers, genomically extended tel- omerase RNA (TR) accumulated at the expense of mature TR, consistent with a role for ZCCHC8 in mediating TR 3′ end targeting to the nuclear RNA exosome. We generated Zcchc8-null mice and found that heterozygotes, similar to −/− human mutation carriers, had TR insufficiency but an otherwise preserved transcriptome. In contrast, Zcchc8 −/− mice developed progressive and fatal neurodevelopmental pathology with features of a ciliopathy. The Zcchc8 brain transcriptome was highly dysregulated, showing accumulation and 3′ end misprocessing of other low-abun- dance RNAs, including those encoding cilia components as well as the intronless replication-dependent histones. Our data identify a novel cause of human short telomere syndromes-familial pulmonary fibrosis and uncover nuclear exosome targeting as an essential 3′ end maturation mechanism that vertebrate TR shares with replication-de- pendent histones. [Keywords: lung disease; ciliopathy; nuclear RNA exosome; RNA processing; telomerase RNA] Supplemental material is available for this article. Received April 19, 2019; revised version accepted August 15, 2019. Telomerase, the specialized ribonucleoprotein that syn- Armanios 2012; McNally et al. 2019). Among IPF families thesizes telomere DNA, has two essential components: with known mutations, half carry mutations in TERT or the telomerase reverse transcriptase, TERT, and, a spe- TR (Armanios et al. 2007; Tsakiri et al. 2007). The rest car- cialized non-coding RNA, TR (also known as TERC), ry mutations in one of five other telomere maintenance which provides the template for telomere repeat addition genes, RTEL1, PARN, NAF1, TINF2, and DKC1 (Alder (Greider and Blackburn 1985, 1987, 1989; Feng et al. 1995; et al. 2013, 2015; Cogan et al. 2015; Stuart et al. Lingner et al. 1997). Mutant telomerase genes have their 2015; Stanley et al. 2016). Four of these seven known mu- most common manifestation in the age-related disease id- tant telomere genes in familial IPF affect TR itself, its iopathic pulmonary fibrosis (IPF) (Armanios et al. 2007; deadenylation, trafficking, or stability as in the case for PARN, NAF1, and DKC1, respectively (Mitchell et al. 1999b; Moon et al. 2015; Stanley et al. 2016). However, 10Present address: Department of Medicine, Massachusetts General Hos- for 50%–60% of IPF families, the genetic etiology is pital, Boston, MA 02114, USA. Corresponding author: [email protected] Article published online ahead of print. Article and publication date are © 2019 Gable et al. This article, published in Genes & Development,is online at http://www.genesdev.org/cgi/doi/10.1101/gad.326785.119. Free- available under a Creative Commons License (Attribution-NonCommer- ly available online through the Genes & Development Open Access cial 4.0 International), as described at http://creativecommons.org/licens- option. es/by-nc/4.0/. GENES & DEVELOPMENT 33:1–16 Published by Cold Spring Harbor Laboratory Press; ISSN 0890-9369/19; www.genesdev.org 1 Downloaded from genesdev.cshlp.org on October 7, 2021 - Published by Cold Spring Harbor Laboratory Press Gable et al. unknown, and within this subset there are individuals This led us to determine preclinical status by measuring with unexplained TR insufficiency and short telomere TR levels in LCLs and we found the proband’s two chil- syndrome features (Stanley et al. 2016). dren also had 50% of control TR levels, while the remain- Here, we used an unbiased genome-wide linkage ap- ing relatives had TR levels similar to healthy controls proach to discover a novel familial IPF disease gene that (Fig. 1B). Telomere length measurement confirmed both is required for TR maturation and telomerase function. of these “low TR” asymptomatic individuals were at Human TR has its own RNA polymerase II transcriptional risk for disease since they had abnormally short telomere unit (Feng et al. 1995); it shares a 3′ end box H/ACA motif length, below the first age-adjusted percentile, and shorter with a subset of non-coding RNAs, but, relative to them, it than the proband (Fig. 1C). These data supported assigning has low abundance (Mitchell et al. 1999a; Chen et al. affected status to the two presymptomatic individuals, 2000; Stanley et al. 2016). Telomere maintenance is vul- and the other family members as unaffected (Fig. 1D). nerable to small reductions in TR levels (Greider 2006; We used these designations to perform a SNP array-based McNally et al. 2019), and TR is haploinsufficient in both genome-wide linkage analysis and identified a single humans and mice (Hathcock et al. 2002; Hao et al. 2005; 17.3-Mb linkage peak on chromosome 12 (1,073,260– Armanios et al. 2009). TR+/− mice show genetic anticipa- 1,246,120) with a maximum log of the odds ratio (LOD) tion with later generations eventually developing short score of 2.99 (Fig. 1E). Whole-genome sequencing identi- telomere phenotypes including bone marrow failure and fied three rare coding variants within this interval in the immunodeficiency (Hathcock et al. 2002; Hao et al. proband (Supplemental Table S1), but only one of them 2005; Armanios et al. 2009; Wagner et al. 2018). The deter- fell in an RNA-related gene, ZCCHC8. The variant, minants of vertebrate TR integrity are incompletely un- c.C557T predicted a p.P186L missense, had not been re- derstood in part because the TR sequence is divergent, ported in >140,000 individuals in the databases (Supple- and different organisms have adapted distinct posttran- mental Table S1) and segregated with both the IPF and scriptional processing strategies (Chen et al. 2000; Podlev- low TR phenotypes (Fig. 1D). In a multi-species align- sky and Chen 2016). Tetrahymena thermophila TR, for ment, p.P186 fell in an unstructured, highly conserved example, is transcribed by RNA polymerase III and relies domain of ZCCHC8 (Fig. 1F; Supplemental Fig. S1). We on runs of T’s for transcriptional termination (Greider and screened 42 other genetically uncharacterized families Blackburn 1989). S. pombe, on the other hand, uses a mod- with pulmonary fibrosis and other short telomere syn- ified spliceosome-dependent cleavage mechanism as an drome phenotypes but did not identify additional initial 3′ end processing step (Box et al. 2008; Coy et al. ZCCHC8 variants, suggesting these mutations are rare 2013). Vertebrate TR is transcribed as a longer RNA (1 of 43, 2%), and consistent with a locus heterogeneity (Feng et al. 1995; Goldfarb and Cech 2013), and a subset for short telomere syndromes. of these extended forms, the short extended oligoadeny- lated ones, were recently found to be precursors for the ZCCHC8P186L is a loss-of-function mutation mature functional TR (Roake et al. 2019). While TR’s deg- radation has been linked to the RNA exosome in some We first tested the functional effects of ZCCHC8P186L and cell-based systems (Nguyen et al. 2015; Tseng et al. found that all three mutation carriers had 50% lower 2015), the mechanisms by which a longer extended TR ZCCHC8 protein levels compared with controls, includ- matures into a shorter, functional RNA are not known. ing noncarrier relatives (LCLs; P = 0.038, Mann-Whitney We report here mutant ZCCHC8, the zinc finger U-test) (Fig. 2A; antibody validation in Supplemental CCHC-type domain containing 8 protein, and a compo- Fig. S2A). Low protein levels were also seen in the pro- nent of the nuclear exosome targeting complex (NEXT) band’s primary skin fibroblasts and were specific to (Lubas et al. 2011), is a cause of familial IPF. We show ZCCHC8 as the levels of the other two NEXT complex that ZCCHC8 is required for TR maturation and uncover proteins, RBM7 and SKIV2L2, were normal (Fig. 2B; two distinct RNA dysregulation
Details
-
File Typepdf
-
Upload Time-
-
Content LanguagesEnglish
-
Upload UserAnonymous/Not logged-in
-
File Pages17 Page
-
File Size-