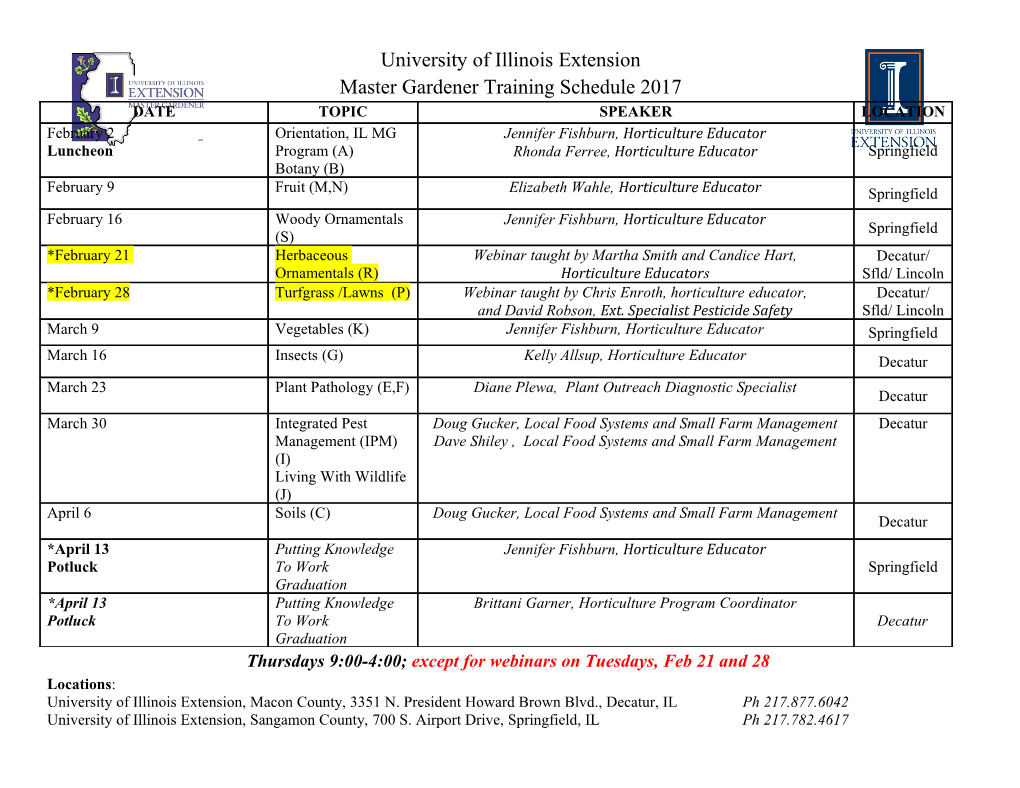
Chapter 2 The .xternal .n8ironment CHAPTER 2 THE EXTERNAL ENVIRONMENT Prepared for the Course Team by Patricia Ash 0.1 Introduction Before we can understand the phenotypic and genotypic adaptations of vertebrates for life in their environment we need to examine the physical characteristics of the environment. Vertebrates live both on land and in water so we need to study the thermal characteristics of each, and to understand the processes of heat exchange. We are especially interested in temperature because the rates of all metabolic processes are temperature dependent. As temperature decreases, enzyme activity usually decreases and so do the rates of most other chemical reactions. The highest temperature available for vertebrate metabolism is restricted by the risk of denaturation of proteins and disruption of membrane structure at temperatures greater than about 38°C. The body temperature of vertebrates represents a balance between heat gained either from metabolism or the environment and heat lost to the environment. Animal physiologists are therefore glucose particularly interested in thermal exchange. Metabolism CYTOSOL glycerol generates heat, and in endotherms this heat is used to maintain body temperature. Therefore, factors affecting metabolic rate alanine pyruvate have been much studied. Until recently most of this research was carried out in a laboratory, but techniques have been developed and refined that enable measurements of body acetyl CoA fatty acid temperature and metabolic rate to be carried out on animals in the field. aspartate 4C 6C MITOCHONDRIAL Nearly all vertebrates depend on oxygen for maintenance MATRIX of aerobic metabolism, and hence generation of ATP, in tissues. TCA cycle The highest yields of ATP per mole of substrate, are obtained glutamate from aerobic catabolism of fuel molecules. Catabolic biochemical pathways convert fuel molecules such as fatty acids and glucose into acetyl coenzyme A (acetyl CoA) 2CO2 2H O (Figure 2.1). Long-chain fatty acids are converted into 2 2-carbon fragments by oxidation, ending in formation 4H + of acetyl CoA. Glucose is converted into pyruvate by the O 2 glycolytic pathway in the cytosol; in turn, pyruvate is electron transport chain INNER converted into acetyl CoA. Acetyl CoA enters the tricarboxylic MITOCHONDRIAL acid (TCA) cycle, combining with the 4-carbon oxaloacetate MEMBRANE to form citrate, the 6-carbon intermediate that is progressively 2H 2 O decarboxylated and oxidized by the TCA cycle. Deamination of certain amino acids results in formation of TCA cycle Figure 2.1 Reactions of oxygen and hydrogen intermediates that are fed in at specific stages in the cycle. atoms during catabolism. Note the entry points for Each full turn of the TCA cycle results in the loss of two fuel oxidation of glucose, fatty acids and certain amino acids. The final stage of catabolism, the electron molecule-derived carbon atoms as CO2 . transport chain, requires oxygen. Overall, for every Hydrogen ions removed from TCA cycle intermediates are taken molecule of acetyl CoA that enters the cycle, two up by the coenzymes, NAD and FAD. Reduced coenzymes, molecules of water are split and two molecules of NAD.2H and FAD.2H, produced during the TCA cycle, are carbon dioxide are produced. Note : ATP generation is oxidized by means of the electron transport chain in the inner not shown in this figure. 01 S324 Book 2 .n8ironmental Physiology mitochondrial membrane. Most ATP synthesis occurs at this stage, powered ultimately by energy released during electron transfer. As oxygen is the final electron acceptor in the electron transport chain, if oxygen is in short supply, the rate of aerobic catabolism and generation of ATP plummets. Depletion of oxygen in tissues, a situation known as hypoxia, results initially in a switch to anaerobic catabolism of glucose, but the yield of ATP per glucose molecule is low, and high levels of the product – lactate – cause problems. In this chapter we will study the relevant aspects of the physics of gases, and examine the situation at high altitude where oxygen is available, but is in relatively short supply. We will study the implications of Fick’s law, which brings together the physical factors that affect the rate of diffusion of gases, and discuss how oxygen is transferred to respiring cells. You should find that the physics explained in Section 2.4 provides useful background for your study of Chapter 6. Extremes of temperature and hypoxia outside normal ranges elicit special biochemical processes called stress responses . Stress responses at the molecular level include those of glycoprotein or protein receptor molecules, either in the cell membrane or cytosol, that detect signals from the environment. Inside the cell, the signal may elicit transcription of specific genes or activation of enzymes, in particular kinases, and often both of these processes. We begin our study of molecular responses to environmental stress in Section 2.8. We end Chapter 2 with an account of the comparatively new field of evolutionary physiology. Study of evolutionary adaptation requires DNA sequence analysis for determination of evolutionary relationships between species. Accurate phylogenetic trees for animal groups are required for distinguishing between phylogenetic characters and features that are adaptive in particular species. If a mutation in DNA is expressed as an altered protein, and is beneficial in a particular environment, it persists and spreads in a population. The importance of the link between animals’ responses to environmental signals at the molecular level and evolutionary adaptation cannot be over-emphasized. 0.0 Thermal characteristics of the e:ternal en8ironment The Sun is the major source of heat energy for the surface of the Earth. The surface temperature of the Earth is the result of the balance between the incoming short-wave radiation from the Sun, and re-radiation of heat energy to space, at a longer wavelength. I Why is energy re-radiated by the Earth at a longer wavelength than that of the short-wavelength radiation that is absorbed from the Sun? The wavelength of radiated energy from a body is related to its temperature – the higher the temperature, the shorter the wavelength. The temperature of the Earth is less than that of the Sun. Therefore, re-radiated energy from the Earth is at a longer wavelength than that absorbed from the Sun. 00 Chapter 2 The .xternal .n8ironment Figure 2.2 shows the electromagnetic spectrum with energy scales in joules 2 20 increasing from left to right and wavelength decreasing from left to right. Note − m that the shorter the wavelength of electromagnetic radiation, the higher the W / ) 15 6 associated energy. If sunlight is split into a spectrum, and the amount of energy 0 1 at different wavelengths is plotted on a graph, a peak occurs at a wavelength of × ( 10 about 500 nm (Figure 2.3a). The temperature of the Sun near its surface is y g around 6000 K. For an object at a temperature of about 300 K ( = 27°C, the r 6000K ene surface temperature of most land mammals), the peak in emitted energy occurs 5 ed at a much longer wavelength, about 15 µ m (Figure 2.3b). The temperature of the tt Earth is about 255 K. emi 0 500 1500 2500 3500 wavelength/nm (a) 2 4 6 8 10 12 14 16 4 2 − − − − − − − − 30 10 10 10 10 10 10 10 10 10 10 3 × 3 × 3 3 × 3 × 3 × 3 × 3 × 3 × 3 × 3 × λ /m v microwaves i ultravioletgamma-rays s 25 i b radio waves infrared l X-rays e 2 10 –30 10 –28 10 –26 10 –24 10 –22 10 –20 10 –18 10 –16 10 –14 10 –12 10 –10 E /J − 20 m W y/ g r 15 ene t t w w e e 300K ed u u nge nge ed ed l een l een tt a r a iole r r iole r b ello b ello r r v g v g y y o o 10 emi 3.04.05.0 E /10 –19J 5 Figure 2.2 The electromagnetic spectrum with photon energy in joules and wavelength, λ , in metres. Note that because the range of photon energies is so large, we have used a power of ten scale; the energy changes by a factor of ten for each 0 10 20 30 40 50 60 70 80 wavelength/ µ m division along the scale. Note also that energy increases from left to right. (b) Figure 2.3 Emission curves for Figure 2.4 (overleaf) summarizes the gains and losses of heat derived from (a) the Sun at 6000 K and incoming solar radiation for the Earth’s atmosphere and surface. Approximately (b) a body at 300 K. 49% of the radiation energy from the Sun that falls on the outer atmosphere reaches the surface directly and is absorbed. Of the other 51%, 31% is scattered by the atmosphere and reflected from the surface and cloud layers, and 20% is absorbed by the atmosphere as long-wavelength radiation. The energy absorbed by the ground/ocean heats the Earth’s surface. The warm surface of the Earth radiates long-wave energy (heat) into the atmosphere (R e ), and also loses heat to the atmosphere by convection and evaporation. In turn the surface absorbs infrared radiation from the atmosphere. These processes of energy loss and gain by the Earth’s surface are balanced, so that there is no overall long-term heat gain or loss by the Earth’s surface. As air has a low specific heat, a small amount of heat produces a relatively large change in temperature. However, air has a low thermal conductivity, which means that heat is not conducted rapidly, so large thermal gradients can build up.
Details
-
File Typepdf
-
Upload Time-
-
Content LanguagesEnglish
-
Upload UserAnonymous/Not logged-in
-
File Pages48 Page
-
File Size-