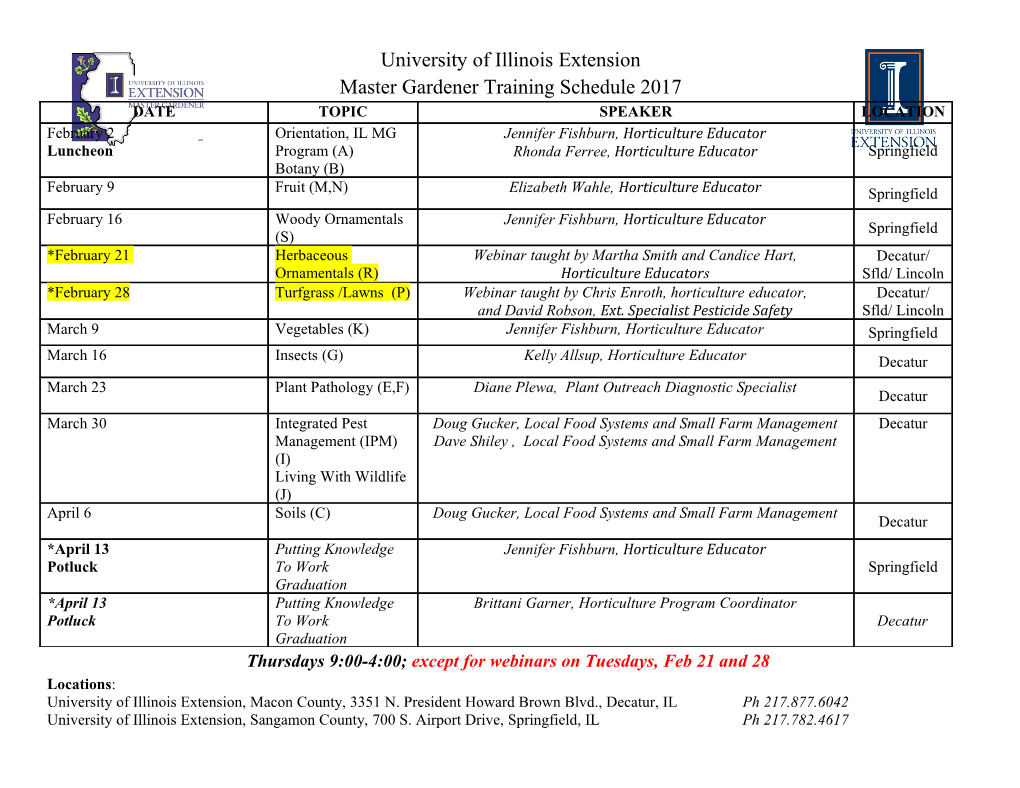
energies Review Nanostructured p-Type Semiconductor Electrodes and Photoelectrochemistry of Their Reduction Processes Matteo Bonomo and Danilo Dini * Department of Chemistry, University of Rome “La Sapienza”, 00139 Rome, Italy; [email protected] * Correspondence: [email protected]; Tel.: +39-06-4991-3335 Academic Editor: Peter J. S. Foot Received: 1 April 2016; Accepted: 6 May 2016; Published: 16 May 2016 Abstract: This review reports the properties of p-type semiconductors with nanostructured features employed as photocathodes in photoelectrochemical cells (PECs). Light absorption is crucial for the activation of the reduction processes occurring at the p-type electrode either in the pristine or in a modified/sensitized state. Beside thermodynamics, the kinetics of the electron transfer (ET) process from photocathode to a redox shuttle in the oxidized form are also crucial since the flow of electrons will take place correctly if the ET rate will overcome that one of recombination and trapping events which impede the charge separation produced by the absorption of light. Depending on the nature of the chromophore, i.e., if the semiconductor itself or the chemisorbed dye-sensitizer, different energy levels will be involved in the cathodic ET process. An analysis of the general properties and requirements of electrodic materials of p-type for being efficient photoelectrocatalysts of reduction processes in dye-sensitized solar cells (DSC) will be given. The working principle of p-type DSCs will be described and extended to other p-type PECs conceived and developed for the conversion of the solar radiation into chemical products of energetic/chemical interest like non fossil fuels or derivatives of carbon dioxide. Keywords: semiconductor; photoelectrochemistry; p-type; reduction; dye-sensitized solar cell; solar fuels; CO2 reduction; metal oxides; metal chalcogenides 1. Introduction The possibility of preparing nanostructured semiconductors of inorganic nature [1] in a controlled fashion allows the creation of systems with elevated chemical and physical stability which are endowed with open morphology and internal electrical transport. Such an achievement led to a rapid development of dye sensitized solar cells (DSCs) with efficiencies in the order of 10% [2–5], a value representing an impressive record if one takes into account that DSCs at their infancy in the late sixties displayed overall conversion efficiencies not exceeding 1% [6]. In the nanoporous version inorganic semiconductors present a lower intrinsic conductivity with respect to their bulk counterparts, and lack the presence of an internal electric field due to the absence of a space charge layer due to charge separation [1]. Compared to bulk semiconductors, the relatively lower electrical conductivity of nanostructured semiconductors is mainly the consequence of frontier molecular orbitals which do not extend over the whole nanostructure thus impeding the delocalization of the electronic charge carriers [7]. Charge transport through such nanostructures occurs per percolation between sub-nanostructures and is based on the phenomenon of electron hopping between localized states with discrete energies [1]. Therefore, the localized states associated with shallow and deep traps as well as surface states, are particularly important in nanostructured semiconductors since both transport and the formation of the electrochemical double layer involve the occupation of such states with isolated energy levels [8]. At the morphological level the surface of nanostructured systems is mesoporous with pores sizes in the order of few nanometers (Figure1)[9]. Energies 2016, 9, 373; doi:10.3390/en9050373 www.mdpi.com/journal/energies Energies 2016, 9, 373 2 of 32 Energies 2016, 9, 373 2 of 32 Energies 2016, 9, 373 2 of 32 Figure 1. SEM image showing the mesoporous surface morphology of a nickel oxide cathode for Figure 1. SEM image showing the mesoporous surface morphology of a nickel oxide cathode for FigureDSC, which 1. SEM has image been showing deposited the mesoporousvia sintering surface of nanoparticles morphology with of a nickeldiameter oxide values cathode comprised for DSC, DSC, which has been deposited via sintering of nanoparticles with diameter values comprised whichbetween has 30 been and 90 deposited nm (evidenced via sintering in yellow). of nanoparticles Reproduced with with diameter permission values from comprised reference [9]. between 30between and 90 30 nm and (evidenced 90 nm (evidenced in yellow). in Reproducedyellow). Reproduced with permission with permission from reference from reference [9]. [9]. This feature leads to a considerable increase (about 1000 times) of the surface area in This feature leads to a considerable increase (about 1000 times) of the surface area in comparisonThis feature to compact leads to surfaces, a considerable and, in increase case of nanostructured (about 1000 times) electrodes, of the surface this brings area inabout comparison also the comparison to compact surfaces, and, in case of nanostructured electrodes, this brings about also the tocorresponding compact surfaces, increase and, of in the case contact of nanostructured area between electrodes, the electrode this brings and electrolyte. about also The the correspondinglatter fact will corresponding increase of the contact area between the electrode and electrolyte. The latter fact will increasegenerally of have the contact very favourable area between effects the on electrode the kinetics and electrolyte. of the processes The latter of charge fact will transfer generally that have are generally have very favourable effects on the kinetics of the processes of charge transfer that are verylocalized favourable on the effects surface on the of kinetics mesoporous of the processeselectrodes of chargeas evidenced transfer thatby the are localizeddirect proportionality on the surface localized on the surface of mesoporous electrodes as evidenced by the direct proportionality ofbetween mesoporous the current electrodes density as evidenced and electrode by the thickness direct proportionality in both dark between conditions the current(Figure density2) [10] andand between the current density and electrode thickness in both dark conditions (Figure 2) [10] and electrodeunder illumination thickness in(Figure both dark 3) [11]. conditions (Figure2)[10] and under illumination (Figure3)[11]. under illumination (Figure 3) [11]. Figure 2. Cyclic voltammetries of mesoporous NiO cathodes at three different values of electrode Figure 2. Cyclic voltammetries of mesoporous NiO cathodes at three different values of electrode thickness ll. J Jand E representE the current density and the applied potential, respectively. Black trace: l thickness l.. J andand E representrepresent the the current current density density and andthe applied the applied potential, potential, respectively. respectively. Black trace: Black l trace:= 0.4 μlm;= 0.4 redµ mtrace:; red l trace: = 1.8 μl =m; 1.8 greenµm; greentrace: trace:l = 2.7l μ=m. 2.7 NiOµm. is NiOthe electroactive is the electroactive species species which = 0.4 μm; red trace: l = 1.8 μm; green trace: l = 2.7 μm. NiO is the electroactive species which undergoes the solid state redox processes NiOx(OH)n(H2O)p → NiOx(OH)n+1(H2O)p−1 + e− + H+ for the´ which undergoes the solid state redox processes NiOx(OH)n(H2O)p Ñ NiOx(OH)n+1(H2O)p´1 + e undergoes the solid state redox processes NiOx(OH)n(H2O)p → NiOx(OH)n+1(H2O)p−1 + e− + H+ for the oxidation+ peak recorded at lower electrical potential, and NiOx(OH)n+1(H2O)p−1 → + H for the oxidation peak recorded at lower electrical potential, and NiOx(OH)n+1(H2O)p´1 Ñ oxidation peak recorded at lower electrical potential, and NiOx(OH)n+1(H2O)p−1 → NiOx(OH)n+2(H2O)p−2 + e− +´ H+ for+ the oxidation peak determined at higher electrical potential. NiOx(OH)n+2(H2O)p´2 + e + H for the oxidation peak determined at higher electrical potential. NiOx(OH)n+2(H2O)p−2 + e− + H+ for the oxidation peak determined at higher electrical potential. Electrolyte: 0.2 M KCl, 0.01 M KH2PO4 and 0.01 M K2HPO4 in distilled water. Scan rate: 40 mV∙´s−11. Electrolyte: 0.2 M KCl, 0.01 M KH2PO4 and 0.01 M K2HPO4 in distilled water. Scan rate: 40 mV¨ s . Electrolyte: 0.2 M KCl, 0.01 M KH2PO4 and 0.01 M K2HPO4 in distilled water. Scan rate: 40 mV∙s−1. AdaptedAdapted fromfrom referencereference [ [10].10]. Adapted from reference [10]. Energies 2016, 9, 373 3 of 32 EnergiesEnergies 2016 2016, 9, 373, 9, 373 3 of3 of 32 32 Figure 3. jV characteristic curves of two p‐DSCs differing for the thickness (l) of the NiO Figure 3.3.jV jVcharacteristic characteristic curves curves of two of p-DSCstwo p‐ differingDSCs differing for the thickness for the (lthickness) of the NiO (l) photocathode. of the NiO Sensitizerphotocathode. is erythrosine Sensitizer B. is Electrolyte: erythrosine 0.1B. Electrolyte: M I , 1.0 M 0.1 LiI M I in2, 1.0 acetonitrile. M LiI in acetonitrile. Incident lightIncident intensity: light photocathode. Sensitizer is erythrosine B. Electrolyte:2 0.1 M I2, 1.0 M LiI in acetonitrile. Incident light intensity:´2 1000 W∙m−2. The radiation source is sun simulator with Air Mass 1.5. Photoelectrode 1000intensity: W¨ m 1000. The W∙ radiationm−2. The sourceradiation is sunsource simulator is sun with simulator Air Mass with 1.5. Air Photoelectrode Mass 1.5. Photoelectrode electroactive electroactive area2 is 4 × 4 mm2. Adapted from reference [11]. areaelectroactive is 4 ˆ 4 mm area .is Adapted 4 × 4 mm from2. Adapted reference from [11
Details
-
File Typepdf
-
Upload Time-
-
Content LanguagesEnglish
-
Upload UserAnonymous/Not logged-in
-
File Pages32 Page
-
File Size-