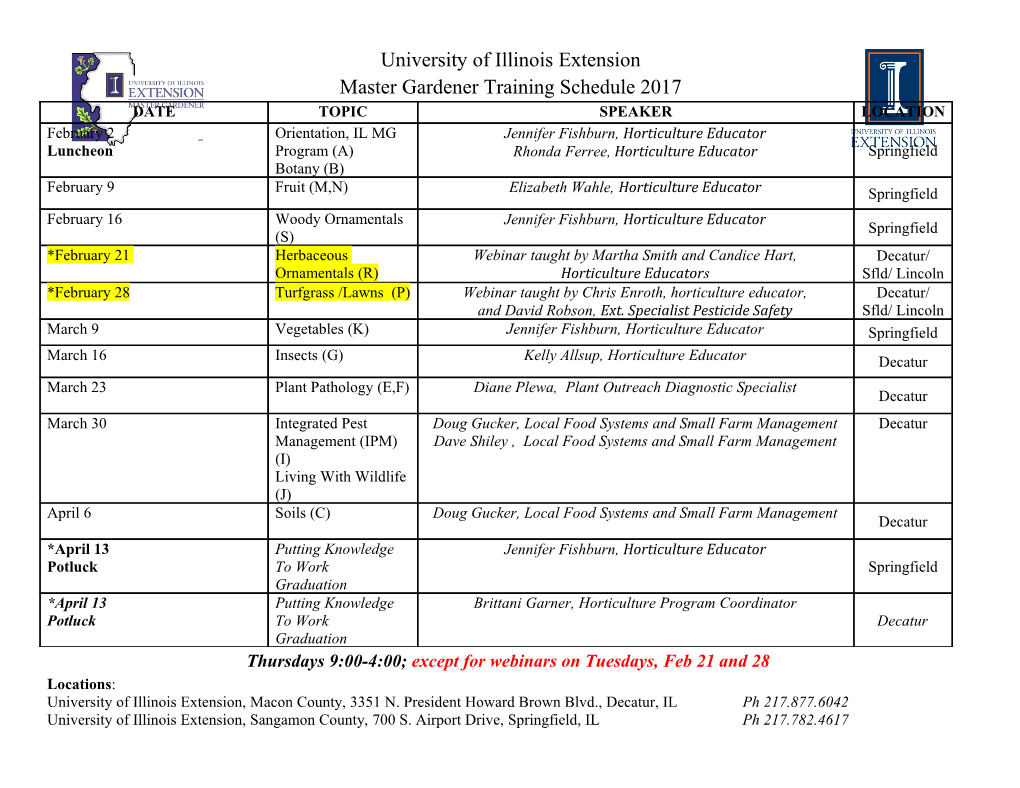
RESEARCH ARTICLE The Magnetosphere‐Ionosphere Electron Precipitation 10.1029/2019JA026589 Dynamics and Their Geospace Consequences Key Points: • Simulated magnetospheric During the 17 March 2013 Storm dynamics depends on the pattern George V. Khazanov1 , Margaret W. Chen2 , Colby L. Lemon2 , and David G. Sibeck1 and intensity of electrons precipitating into the atmosphere 1NASA Goddard Space Flight Center, Greenbelt, MD, USA, 2The Aerospace Corporation, El Segundo, CA, USA • Precipitating electron energy fluxes enhanced by multiple atmospheric reflections affect ionospheric conductance Abstract During geomagnetic storms and substorms, the magnetosphere and ionosphere are strongly • Reduced electric field shielding at coupled by precipitating magnetospheric electrons from the Earth's plasma sheet and driven by both low L can lead to an increase in magnetospheric and ionospheric processes. Magnetospheric wave activity initiates electron precipitation, modeled ring current energy content and the ionosphere and upper atmosphere further facilitate this process by enhancing the value of precipitated energy fluxes via connection of two magnetically conjugate regions and multiple atmospheric reflections. This paper focuses on the resulting electron energy fluxes and affiliated height‐integrated Correspondence to: fl G. V. Khazanov, Pedersen and Hall conductances in the auroral regions produced by multiple atmospheric re ections during [email protected] the 17 March 2013 geomagnetic storm and their effects on the inner magnetospheric electric field and ring current. Our study is based on the magnetically and electrically self‐consistent Rice Convection ‐ fi Citation: Model Equilibrium of the inner magnetosphere with SuperThermal Electron Transport modi ed electron Khazanov, G. V., Chen, M. W., Lemon, energy fluxes that take into account the electron energy interplay between the two magnetically conjugate C. L., & Sibeck, D. G. (2019). The ionospheres. SuperThermal Electron Transport‐modified energy flux in the Rice Convection ‐ magnetosphere ionosphere electron ‐ fi precipitation dynamics and their Model Equilibrium leads to a signi cant difference in the global conductance pattern, ionospheric electric geospace consequences during the 17 field formation, Birkeland current structure, ring current energization and its energy content, subauroral March 2013 storm. Journal of polarization drifts intensifications and their spatial locations, interchange instability redistribution, and Geophysical Research: Space Physics, 124. https://doi.org/10.1029/ overall energy interplay on the global scale. 2019JA026589 Received 1 FEB 2019 1. Introduction Accepted 11 JUN 2019 Accepted article online 26 JUN 2019 The importance of ionospheric conductivity in coupling magnetospheric and ionospheric plasmas has been recognized since the early days of space science. Wolf (1970) and Vasyliunas (1970) used the ionospheric Ohm's law to calculate the electric field distribution governing magnetospheric plasma circulation. The central importance of ionospheric electrodynamics and conductivity in governing magnetosphere‐ ionosphere‐thermosphere dynamics is evident across a broad range of solar terrestrial science topics: for example, thermospheric responses to geomagnetic storms (Wang et al., 2008), conductivity correlations with dayside field‐aligned currents (Ohtani et al., 2005), global magnetosphere configuration (Ridley et al., 2004), substorm dynamics (Raeder et al., 2001), ring current dynamics (Chen et al., 2015; Ebihara et al., 2004), sub- auroral polarization drifts (SAPS; Anderson et. al., 1993; Zheng et al., 2008; Huba et al., 2017; Mishin et al., 2017), and plasma sheet fast‐flow dynamics (Zhang et al., 2012). Ionospheric conductance depends strongly on particle precipitation into the atmosphere. Both ions and electrons precipitate in the aurora; however, the average integral number flux of the precipitating auroral ions is typically 1 to 2 orders of magnitude less than that of the precipitating auroral electrons (Hardy et al., 1989). Simulations of Chen et al. (2019) of electron precipitation (due to wave scattering) and ion precipitation (due to field line curvature) during a large storm showed that precipitating electrons are the dominant contributor to auroral conductance. Therefore, the electron precipitating flux requires careful examination. Chen et al. (2015) noted “The simulated stormtime ring current energization can vary significantly depending on the ionospheric conductance and electron loss model used. Thus, it is important to incorporate realistic descriptions of ionospheric conductance and electron losses in inner magnetospheric models.” Early simulations of diffuse electron auroral precipitation assumed strong pitch angle scattering ©2019. American Geophysical Union. everywhere with perhaps a constant fraction of backscatter (Chen & Schulz, 2001; Fontaine & Blanc, This article has been contributed to by 1983; Wolf et al., 1982). Chen, Lemon, Orlova, et al. (2015) showed that strong pitch angle scattering caused US Government employees and their fl work is in the public domain in the electron losses that were too rapid to account for observed trapped electron uxes at Geosynchronous orbit. USA. Recent models of Chen et al. (2019), Chen, Lemon, Orlova, et al. (2015), and Yu et al. (2016) that include KHAZANOV ET AL. 1 Journal of Geophysical Research: Space Physics 10.1029/2019JA026589 more realistic electron losses of pitch angle scattering with chorus waves have been able to reproduce rea- sonably well observed diffuse precipitating electron energy fluxes near dawn during storm events. However, further work is needed to characterize global patterns of electron precipitation. During geomagnetic storms and substorms, the magnetosphere and ionosphere are strongly coupled by pre- cipitating magnetospheric electrons from the Earth's plasma sheet. As has been shown by Khazanov et al. (2014) about 15–40% of the total aurora energy returns back to the magnetosphere and the conjugate iono- sphere. Collisionless processes dominate in the inner magnetosphere, whereas collisional interactions are important in the ionosphere, and Khazanov et al. (2018) have emphasized that first‐principle simulations of precipitating electron fluxes in both regimes are required to understand spatial and temporal variations of ionospheric conductance and related electric fields. As discussed by Khazanov et al. (2015), Khazanov, Glocer et al. 2016, 2017a), the first step in such simulations is initiation of electron precipitation from the Earth's plasma sheet via wave‐particle interactions (WPIs) into both magnetically conjugate points (e.g., as is simulated by Chen, Lemon, Guild, et al., 2015). The second step is to account for multiple atmospheric reflections of electrons between the ionosphere and magnetosphere at the two magnetically conjugate points, as treated in the SuperThermal Electron Transport (STET) model (Khazanov et al., 2015, 2017a, 2017b, 2018; Khazanov, Glocer, et al., 2016; Khazanov, Himwich, et al., 2016). This paper focuses on the resulting electron energy fluxes and affiliated height‐integrated Pedersen and Hall conductances in the auroral regions produced by multiple atmospheric reflections during the large 17 March 2013 geomagnetic storm and their effects on the inner magnetospheric electric field and ring current. Our study is based on the Aerospace version of the magnetically and electrically self‐consistent Rice Convection Model‐Equilibrium (RCM‐E) of the inner magnetosphere (Chen, Lemon, Guild, et al., 2015), with STET‐modified electron energy fluxes that take into account the electron energy interplay between the two magnetically conjugate ionospheres. 2. Role of MI Coupling in Electron Precipitation Formation In the aurora, precipitating electrons driven by WPI in the Earth's plasma sheet collide with atmospheric molecules, lose energy, and produce secondary electrons (SEs) via impact ionization. The precipitating and SEs can also reflect back into the magnetosphere and then undergo multiple reflections at the magnetic conjugate points. As was shown by Khazanov et al. (2015–2018), these processes greatly influence the total precipitating flux at the upper ionospheric boundary (around about 800 km). The resultant populations of secondary and primary electrons undergo both elastic and inelastic collisions with the neutral atmosphere, cascading toward lower energies and reflecting back to the magnetosphere. Those electrons reflected upward from the ionosphere can be trapped in the magnetosphere if they scatter out of the loss cone via cou- lomb collisions with the cold plasma or through interactions with different kinds of plasma waves. In situ measurements of the precipitating electron energy flux spectra in the ionosphere naturally include all these effects. However, the physics of multiple reflections and scattering has been missing in aurora global mod- eling research, and in this study we add this capability to RCM‐E simulations and examine the effects on inner magnetospheric plasma transport and the electric and magnetic fields. The STET code models all sources and collisional processes of electrons as they travel along a magnetic field line through the magnetosphere and ionosphere (Khazanov et al., 2015; Khazanov, Glocer, et al., 2016; Khazanov, Himwich, et al., 2016; Khazanov et al., 2017b) with participation of both magnetically conjugate regions. It simulates nonsteady formation of electron distribution functions kinetically along 1‐D in space and 2‐D in velocity
Details
-
File Typepdf
-
Upload Time-
-
Content LanguagesEnglish
-
Upload UserAnonymous/Not logged-in
-
File Pages20 Page
-
File Size-