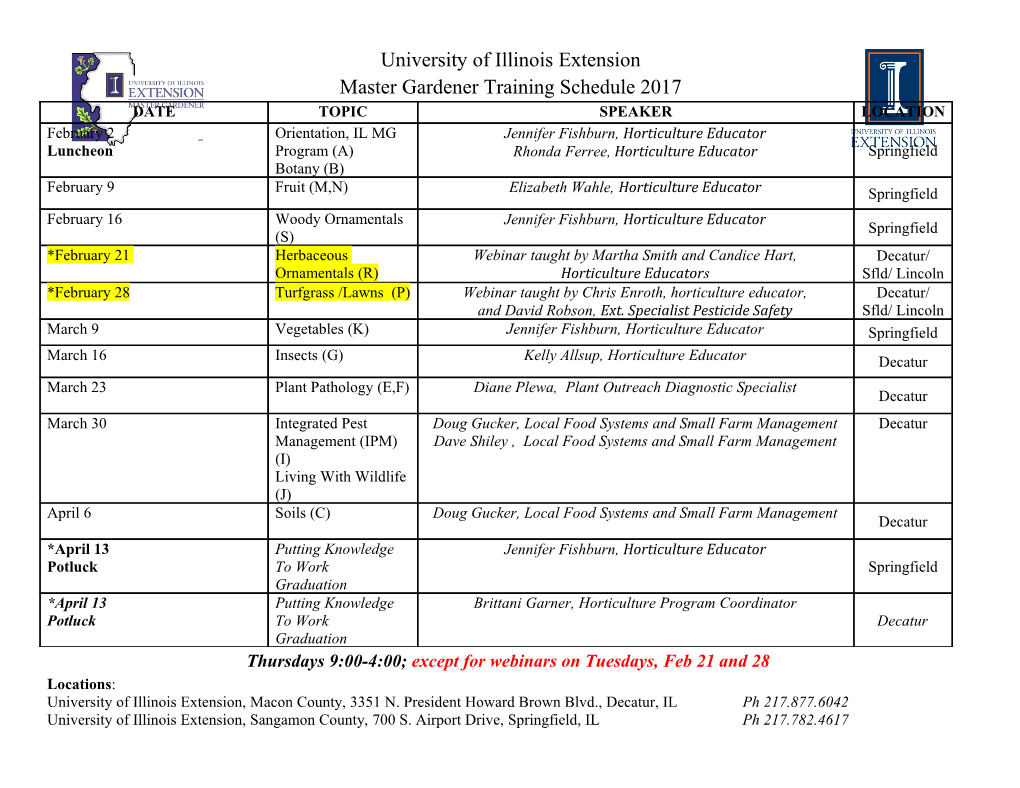
The Pennsylvania State University The Graduate School Department of Chemistry INVESTIGATING RNA FOLDING AND ADAPTATION IN CELLULAR CONDITIONS A Dissertation in Chemistry by Kathleen A. Leamy © 2018 Kathleen Leamy Submitted in Partial Fulfillment of the Requirements for the Degree of Doctor of Philosophy August 2018 The dissertation of Kathleen A. Leamy was reviewed and approved* by the following: Philip C. Bevilacqua Distinguished Professor of Chemistry and Biochemistry and Molecular Biology Dissertation Advisor Chair of Committee Joseph A. Cotruvo Assistant Professor of Chemistry Louis Martarano Career Development Professor Frank L. Dorman Associate Professor Biochemistry and Molecular Biology Xin Zhang Assistant Professor of Chemistry Assistant Professor of Biochemistry and Molecular Biology Paul Berg Early Career Professorship in the Eberly College of Science Thomas E. Mallouk Head of the Chemistry Department Evan Pugh Professor of Chemistry, Biochemistry and Molecular Biology, Physics, and Engineering Science and Mechanics *Signatures are on file in the Graduate School ii Abstract RNA is an essential biomolecule that controls many processes in cells by folding into complex three-dimensional structures. Regulation can happen at the transcriptional or translational levels by RNA self-cleavage, ligand binding, or unfolding, to name a few. The folding and structures of these so called ‘functional RNAs’ that adopt tertiary structures has been studied for several decades. These studies have revealed that functional RNAs fold in a hierarchical manner where secondary structures form before tertiary structure, RNA folds over broad energetic landscapes, and RNAs become trapped in stable misfolded, non-functional structures that can last for hours. The majority of these studies have been performed on just a few RNA sequences and in classic solution conditions of 1 M Na+. Although these studies have revealed fundamentals of RNA folding, they have been performed in non-biological solutions and on a low range of sequence diversity. This limits our knowledge of how RNA folds in cells. In cells the ionic conditions are much lower than those typically used in vitro. There is ~ 140 mM K+, 10 mM Na+, 0.5-1.0 mM Mg2+ in eukaryotic cells, and 1.5-2.0 mM Mg2+ in prokaryotic cells. There are also numerous small molecules, including free nucleotides, amino acids, and metabolites, and the biomolecule and organelles contribute 20-40% molecular crowding. Recent efforts have shown that mimicking some of these cellular conditions in vitro affect the folding and function of RNAs. In all chapters of the work herein, I describe the effects of cellular mimics on RNA folding processes, iii including the stability of secondary and tertiary structures, cotranscriptional folding, and RNA adaptation to high temperatures. Several functional RNAs have been shown to fold in a more two-state manner in cellular mimics, but in a multi-state manner in classic in vitro conditions. However, the mechanism behind this two-state folding has been largely uninvestigated. In Chapter Two, I focus on how cooperativity arises in biological crowded and Mg2+ conditions by studying the influence of these conditions on the structure and stability of the secondary and tertiary structures of tRNAphe. I find that cooperativity occurs through both a destabilization of secondary structures and a stabilization of tertiary structure. This is very similar to the folding of proteins, where weak secondary structure and strong tertiary structure drive cooperativity to the native state. Full-length RNAs are typically studied by renaturing a full-length transcript in vitro and watching it fold or unfold. However, in cells the growing transcript folds as it emerges from the polymerase, and the mechanism behind cotranscriptional structure formation is largely unknown. In Chapter Three, I study cotranscriptional structure formation in tRNAphe. I find that in cellular mimics, structures that form cotranscriptionally are weakened compared to in buffer, until a single nucleotide is added and a large increase in transcript stability is observed. This large increase in stability results in cooperative folding to the native state, and only happens when all secondary and tertiary contacts can form. This single nucleotide control of folding is observed both experimentally and computationally, and suggests that nature has selected for minimal structure formation iv in cotranscriptional intermediates until two-state folding to the functional state can be achieved. Organisms grow at a wide range of temperatures, which range from freezing to boiling, and their RNAs need to adopt to fold and function in those extreme environments. Yet, only a few sequences of a type of RNA are typically studied. In Chapter Four, I investigate how tRNAphe adapts to extreme temperatures, and how model transcripts from thermophiles fold under cellular conditions. The data reveals that tRNA adapts to extreme temperature by altering the stability of secondary structures, while nucleotides in tertiary structures are highly conserved. We also show that model transcripts from thermophiles maintain cooperative folding, but only in the most cellular- like solution conditions. This study showed a novel mechanism for functional RNA adaptation. A challenge in the RNA folding community is that there is not a good method for studying thermodynamics in complex solution conditions, such as cell extracts. This has two main causes: (1) Cosolutes and biomolecules interfere with the signal from an RNA of interest, which is normally UV detected at 260 or 280 nm, and (2) Methods to study thermodynamics typically involve heating the sample to denature the RNA, however this will also denature biomolecules in solution, no longer making it cellular-like. In Chapter Five, I describe efforts to develop a method to study RNA thermodynamics in complex cellular mimics that will overcome the above described challenges. Overall, the work presented in this thesis provides insights into how RNAs fold and adapt in cellular conditions, with implications for understanding how nature selects for RNA structures. v TABLE OF CONTENTS LIST OF FIGURES………………………………………………………………………………………………………………x LIST OF TABLES…………………………………………………………………………………………………………..…xv ABBREVIATIONS………………………………………………………………………………………………………….xvii ACKNOWLEDGEMENTS………………………………………………………………………………………………xviii Chapter 1 INTRODUCTION……………………………………………………………………………………….1 1.1 RNA folding pathways and an introduction to cooperativity…………………………..2 1.2 RNA folding in vitro and in vivo…………………………………………….………………………..5 1.3 Designing in vivo-like conditions……………………………………………………………………8 1.4 Influence of in vivo-like conditions on RNA folding and function…………………….9 1.5 Influence of cotranscriptional folding and RNA adaptation…………………………..11 1.6 Transfer RNA maturation and processing…………………………………………………….13 1.7 Thesis objectives…………………………………………………………………………………………14 1.8 References………………………………………………………………………………………………….16 Chapter 2 COOPERATIVE RNA FOLDING UNDER CELLULAR CONDITIONS ARISES FROM BOTH TERTIARY STRUCTURE STABILIZATION AND SECONDARY STRUCTURE DESTABILIZATION……………………………………………………………………………………..………………….23 2.1 Abstract………………………………………………………………………………………………………23 2.2 Introduction………………………………………………………………………………………………..24 2.3 Results and discussion………………………………………………………………………………..28 2.3.1 Effects of Mg2+ and crowder are similar for WT and FL tRNA………..29 2.3.2 Cooperative tRNA folding can be induced by Mg2+-driven tertiary structure stabilization……………………………………………………………………………29 2.3.3 Cooperative tRNA folding can be induced by crowder-driven secondary structure destabilization……………………………………………….……..33 2.3.4 Cooperative and non-cooperative folding are observed on the nucleotide level………………………………………………………….…………………………36 2.3.5 tRNAphe adopts a more compact structure under in vivo-like conditions……………………………………………………………………………………………..43 2.4 Conclusions…………………………………………………………………………………………………47 2.5 Acknowledgements…………………………………………………………………………………….49 2.6 References………………………………………………………………………………………………….49 vi Chapter 3 COTRANSCRIPTIONAL FOLDING OF RNA IS COOPERATIVE UNDER PHYSIOLOGICAL CONDITIONS……………………………………………………………………………….………55 3.1 Abstract……………………………………………………………………………………………………… 55 3.2 Introduction………………………………………………………………………………………………..56 3.3 Results…………………………………………………………………………………………………………59 3.3.1 Effects of cellular conditions on cotranscriptional folding in eukaryotic Mg2+……………………………………………………………………………….…… 60 3.3.2 Effects of cellular conditions on cotranscriptional folding in prokaryotic Mg2+……………………………………………………………………………………64 3.3.3 Cooperativity arises from depopulation of non-native states…………65 3.3.4 Extensions on the 5’ and 3’ end do not affect tRNA core folding cooperativity…………………………………………………………………………………………67 3.3.5 Cooperative cotranscriptional folding is computationally predicted……………………………………………………………………………………………… 70 3.4 Discussion……………………………………………………………………………………………………73 3.5 Conclusions…………………………………………………………………………………………………76 3.6 Acknowledgments……………………………………………………………………………………….77 3.7 References………………………………………………………………………………………………….78 Chapter 4 MOLECULAR MECHANISM FOR FOLDING COOPERATIVITY OF FUNCTIONAL RNAS IN LIVING ORGANISMS…………………………………………………………………………………………83 4.1 Abstract………………………………………………………………………………………………………83 4.2 Introduction………………………………………………………………………………………………..84 4.3 Results…………………………………………………………………………………………………………86 4.3.1 Dilute solution conditions lead to non-cooperative folding with stronger base pairing…………………………………………………………………………….86
Details
-
File Typepdf
-
Upload Time-
-
Content LanguagesEnglish
-
Upload UserAnonymous/Not logged-in
-
File Pages218 Page
-
File Size-