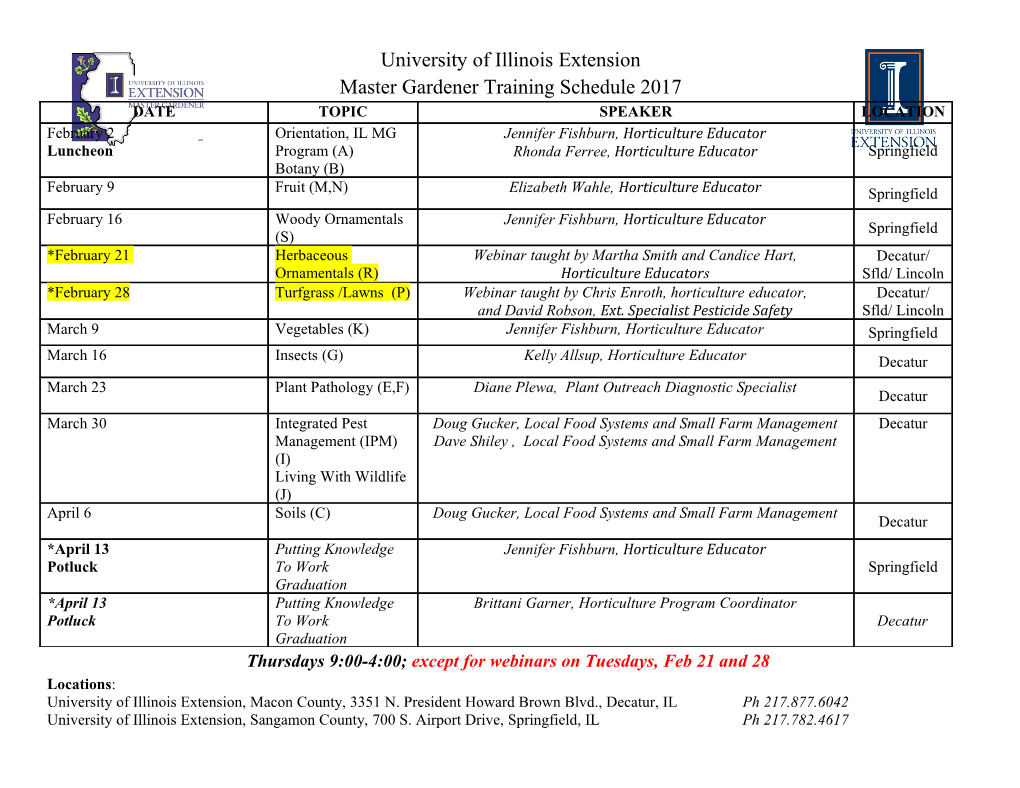
by Dr. Raun Lohry Ortho Vs. Poly Author takes a look at the history and behavior of ortho and polyphosphates. Summary: Reports covering nearly 40 temperature related. Many know first barley. Gilliam and Sample (1968) years of research present strong hand the problem of hydrolysis that studied hydrolysis rates in soils with evidence of the rapidity of phosphate occurs if APP is left to “cook” all different chemical properties to assess hydrolysis. Whether hydrolysis is summer in a tank. Poly content is the relative importance of chemical and complete in a few days or weeks, the reduced and sequestered metals may biological influences. They found a process is fast enough to supply plants fall out leaving residue in the tank (and significant chemical contribution to and roots with sufficient cloudy product). And, once the polys hydrolysis rate. All the observed orthophosphate. are hydrolyzed, the product may not be changes could not be attributed solely hosphorus is required for life. It able to sequester added micronutrients to biological factors. Coarse-textured is the main component of ATP— such as zinc. Dilute APP solutions may soils appeared to hydrolyze PP faster Pthe compound essential for hydrolyze to orthophosphate but water than fine. Hons et al. (1986) also found energy transfer. It is part of a myriad of dilution does not appear to accelerate texture to significantly interact with functions. Plants are generally thought the normal hydrolysis process. other factors to influence rate. to consume only the phosphate in the Adding APP to soil is quite a different Significant interactions expressed were: ortho form. Why then are our modern- matter. Research studies examining the texture x organic matter content, day high polyphosphate fertilizers conversion of condensed phosphates to texture x pH, texture x time, organic effective in overcoming soil orthophosphate report half-lives of less matter x time, pH x soil moisture, pH x phosphorus (P) deficiencies when they than one day to as long as 100 days. A time, and temperature x time. contain large portions of their half-life is the time it takes to convert Dick and Tabatabai (1986) phosphate in the condensed forms – half of the polyphosphate to demonstrated hydrolysis rate principally pyrophosphate and tri- orthophosphate. Some conditions that differences in four soils at three polyphosphate? It’s a question we get influence conversion rate are temperature regimes (Figure 1). Rates asked more and more, especially with temperature, pH, aerobic status, were lower at 50° than at 68° or 86° F. the increased interest in the use of in- biological activity, and minerals. The amount of P hydrolyzed in the furrow starter fertilizers. Liquid polyphosphate converts more three acid soils (Clarion, Webster, Liquid fertilizer began its growth quickly than dry. Water-soluble polys Muscatine) decreased with increasing with orthophosphates. Early in the convert quicker than acid-soluble. chain length although there were no sixties, the Tennessee Valley Authority Researchers have had to take extra care significant differences between pyro- with soil sample storage since (P ) and tri-polyphosphate (P ). (TVA) researched methods to make a 2 3 more concentrated liquid phosphate. polyphosphates convert more rapidly in Chang and Racz (1977) quantified Growers appreciated many of the field-moist soils than air-dried. temperature effects on sodium benefits of liquid fertilizers but there Sodium phosphate research pyrophosphate hydrolysis (Figure 2). was a desire to provide more plant food Sutton and Larsen (1964) studied the Rates increased linearly and increased per gallon of fertilizer. TVA found that hydrolysis rate of radioisotope-labeled about two- to three-fold from 68° to 95° removing bound water from phosphoric sodium pyrophosphate in pot and water F. Tri-polyphosphate hydrolysis was acid boosted the phosphate content cultures. They surmised that hydrolysis greater than pyrophosphate and both from 54% to 70%. This 30% increase to orthophosphate was largely rates were higher in the non-calcareous reduced freight costs and made a whole enzymatic and reported half-lives soil. About 40-70% of the phosphate new series of products possible. Super ranged from 4 to 100 days with the hydrolyzed in 120 hours at 68° F acid was born. Reacting super acid with average being 18. Rates were higher at whereas about 80-95% hydrolyzed in ammonia under controlled conditions higher soil pH values. Hydrolysis 120 hours at 95° F resulted in highly concentrated, easy to proceeded more quickly with higher Minerals may also affect hydrolysis handle, neutralized liquid ammonium biological activity (as measured by CO2 rate. Dick and Tabatabai (1987) polyphosphates (APP). The + evolution). Pyrophosphate was not showed Ca2 , pH, and non-buffered polyphosphates (PP) sequestered converted rapidly in the water culture phosphatase activity to be positively magnesium, iron, and aluminum which and plants absorbed 2.4 times more correlated with hydrolysis rate while pose problems in orthophosphates. orthophosphate. Subsequently, Sutton, percentage of clay, extractable Al3+, These new phosphates could accept et al. (1966) found that and water soluble Mg2+ were negatively non-chelated zinc. pyrophosphatase level, CO2 evolution, correlated. Soil reactions temperature, and uptake were loosely APP correlated. Low temperatures restricted There is a normal hydrolysis of The most commonly applied hydrolysis and therefore P uptake in concentrated APP that is strongly polyphosphate is ammonium Fall 2001 Fluid Journal polyphosphate. There are likely only Table 1. Half-life values (days) for solid and liquid APP in different soils subtle differences between ammonium under aerobic and anaerobic conditions. and sodium phosphate reactions in soils (Mnkeni and Mackenzi, 1987). Solid APP Liquid APP Hashimoto et al. (1969) reported that Aerobic Anaerobic Aerobic Anaerobic APP hydrolysis increased with greater microbial activity and indicated that Alluvial 27.0 9.2 8.7 2.0 the catalytic influence of soil minerals was less with higher APP Sodic 15.0 7.0 5.4 1.8 concentrations. Sample et al. (1979) reported that diammonium phosphate Laterite 12.5 3.9 5.2 1.6 (DAP), triammonium pyrophosphate (TPP), and APP fertilizer additions behave essentially as salts. The NH4+ moved along with the phosphate ions in the form of salt diffusion. High Temperature (F ) concentrations caused the NH4+ to 50 68 86 displace calcium on the exchange CLARION SOIL complex. Displaced calcium 60 precipitated phosphates. Ortho and 40 polyphosphates influenced soil aluminum (Al) and calcium (Ca) by 20 releasing into soil solution and subsequent reprecipitation. Both 0 elements appeared to be reprecipitated in place with added DAP. Sequestration by TPP and APP moved the cations 60 WEBSTER SOIL slightly before complex ion reactions caused precipitation back to insoluble 40 forms. Virtually all movement was in 20 the ortho form (Sample et al., 1979) probably due to the high hydrolysis 0 rate. Hydrolysis was occurring faster than diffusion through the soil column. They calculated hydrolysis half-lives 60 MUSCATINE SOIL for the first two weeks as 8.8 days and for the second two weeks as 15.6 days. 40 Venugopalan and Prasad (1989) also 20 showed that hydrolysis rate was rapid in the beginning and slowed down with 0 incubation time, regardless of soil type. They further demonstrated that liquid POLYPHOSPHATE-P HYDROLYZED (%) HYDROLYZED POLYPHOSPHATE-P CANISTEO SOIL APP hydrolyzed faster than solid APP 60 and that anaerobic conditions (caused 40 by subsequent flooding) accelerated hydrolysis (Table 1). 20 Plant uptake 0 Polyhosphates are widely distributed P P TMP P P P P P among bacteria, blue-green algae, 2 3 5 15 25 35 65 fungi, and algae. Meta- and linear polyphosphates with chain lengths as Figure 1. Effect of temperature on hydrolysis of polyphosphates in soils long as 500 have been isolated from incubated for seven days. microorganisms. Plant available phosphate is mainly controlled by hydrolysis reactions because most P is taken up as orthophosphate. Small amounts may be used as pyrophosphate. Dick and Tabatabai (1986) found that hydrolysis rates were Fall 2001 Fluid Journal faster in non-sterile solutions 100 (A) 100 (B) presumably because corn roots exude 95F 95F enzymes which facilitate the conversion 80 80 68F to orthophosphate. Gilliam (1970) reached similar conclusions in 60 68F 60 41F experiments using 32P. He concluded 40 40 that ortho and pyrophosphate were 41F equally effective in supplying P to P- 20 20 deficient wheat, corn, and barley. P- deficient roots absorbed the 0 0 24 48 72 120 24 48 72 120 pyrophosphate ion per se and also LAKELAND SOIL NEWDALE SOIL rapidly hydrolyzed the PP. Nearly 55% of the P was present in the ortho form after 24 hours. P-deficient wheat roots 100 (C) 100 (D) 95F had 1.6 times as much pyrophosphatase 95F activity as non-deficient roots. (%) HYDROLYZED 80 80 68F 68F 41F Dr. Lohry is agronomist for 60 60 Nutra-Flo Company. 41F 40 40 20 20 0 0 24 48 72 120 24 48 72 120 LAKELAND SOIL NEWDALE SOIL Time (hours) Figure 2. Temperature effect on hydrolysis of water-soluble sodium pyrophosphate and sodium tripolyphosphate. A and B = 200ppm pyrophosphate; C and D = 200ppm tripolyphosphate. Fall 2001 Fluid Journal.
Details
-
File Typepdf
-
Upload Time-
-
Content LanguagesEnglish
-
Upload UserAnonymous/Not logged-in
-
File Pages3 Page
-
File Size-