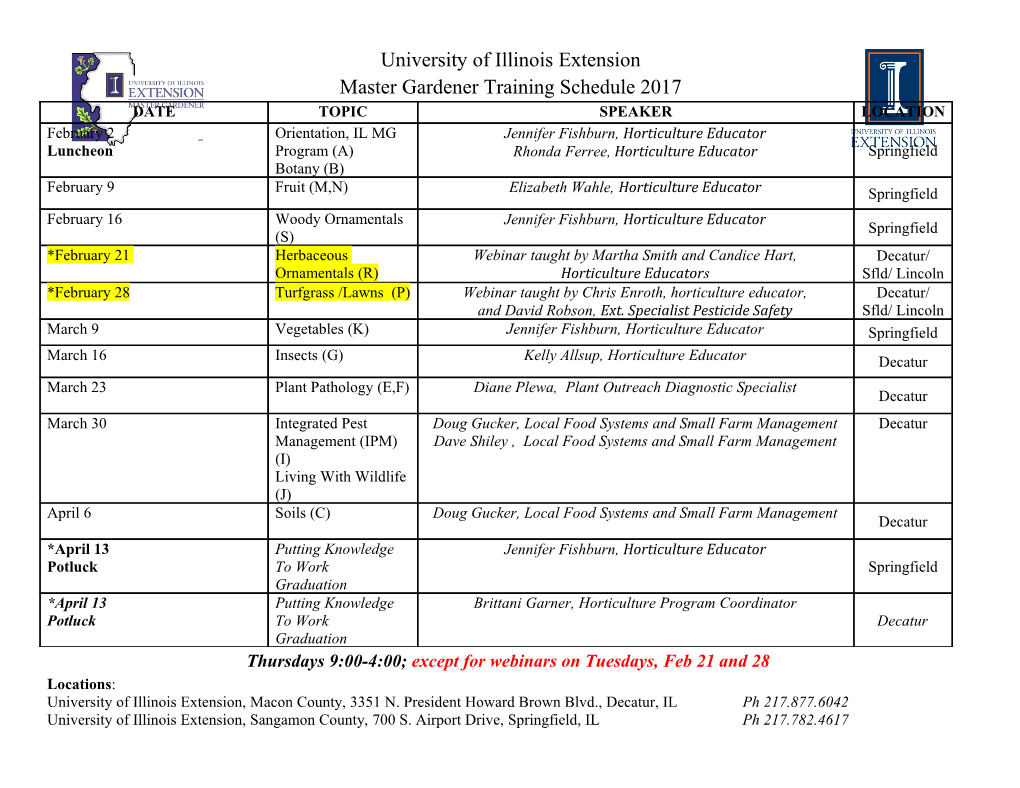
Journal of the Ceramic Society of Japan 110 [2] 86-91 (2002) Paper Transmission Electron Microscopy Study of Defect Structure in Epitaxial SnO2 Rutile Thin Film Toshimasa SUZUKI, Hirotaka WAKABAYASHI, Yuji NISHI and Masayuki FUJIMOTO Taiyo Yuden Co., Ltd., 5607-2, Nakamuroda, Hayuna-machi, Cunma 370-3347 エ ピ タ キ シ ャ ルSnO2ル チ ル 薄 膜 中 の 欠 陥 構 造 の 透 過 型 電 子 顕 微 鏡 研 究 鈴木利 昌 ・若林博孝 ・西 湯二 ・藤本正之 太陽誘電(株), 370-3347群馬県群馬郡榛名町中室田 5607-2 SnO2 thin film heteroepitaxially grown on a rutile (100) TiO2 single crystal substrate exhibits high-density interfacial misfit dislocations and related planar defects. The misfit dislocation network formed on the het- erointerface consists mainly of two types of partial edge dislocations with Burgers vectors of 1/2[101] and 1/2[110], to fully relieve the lattice mismatch, and the dislocations inevitably involve inclined (101) and ver- tical (010) planar defects extending toward the film surface, respectively. Some of the (101) planar defects form wedge-shaped defects, and frequently accompany a single or multiply stacked (101) nanotwin lamellae. Another (011) type of planar defect originating at fused threading dislocations with 1/2[011] Burgers vec- tors is also detected by plan-view observation, showing the same crystallographic feature as (101) planar defects observed in the cross-sectional (010) projection. The dominant relaxation mechanism through par tial edge dislocations suggests the low interfacial energy of the defect plane in the rutile structure, hinder ing the control of high crystallinity in rutile oxide thin films. [Received August 7, 2001; Accepted December 5, 2001] Key-words: SnO2, Defect, Heteroepitaxy,Rutile, Crystallographic shear structure, Misfit dislocation 1. Introduction vectors.9),10) It is of interest that other types of non- Recently advancing thin-film planar technology has stoichiometry-related CSP defects commonly reported in driven an increasing interest in new classes of smaller, high- reduced bulk ceramics or powders, (121), (132), and performance integrated microdevices including oxide (142) ,13)-16) were not observed in low-temperature- materials formed with various elements of the periodic ta processed rutile oxide films, even with oxygen-deficient ble. The oxide materials exhibit various physical properties, compositions, probably due to limited vacancy migration. such as f erroelectric, high-dielectric, piezoelectric, optical, The epitaxial single-crystalline forms, on the other hand, are and superconductive properties, and thus the extensive in- less desirable for gas sensing applications, because of their tegration of such materials based on planar technology will boundary-less structure, but there exist some more attrac provide highly functional devices, where a significant selec tive studies focusing on epitaxial rutile films with the expec- tion of constituent elements and crystallographic structures tation of superior performance based on simplified gas-solid are available. Many obstacles for these recent applications, interactions of single crystal film17) and in-plane valiant however, remain especially in the controllability of oxide structure utilizing the difference between the film and sub thin-film crystal growth.1) Next-generation heteroepitaxial strate symmetry.18) Such epitaxial techniques are naturally multiplayer applications such as artificially structured quan- required for advancing integrated electronic devices and op tum wells and superlattices, and multiplayer optical devices, tical wave guide systems,19),20) and thus the detailed under actually require high controllability of crystal growth and standing of lattice defects closely related to misfit relaxation lattice defects comparable to ordinal semiconductor is essential for acquiring the desired crystallinity. technology.2) The detailed investigations of heteroepitaxial In a previous work, we reported the epitaxial growth, growth and associated defect generation mechanisms are, defect structure and related surface morphology of rutile however, quite few in number compared to those for semi- SnO2 thin films, using transmission electron microscopy, conductor electrical and optical devices, and, in particular, reflection high-energy electron diffraction, and atomic force the basic crystallographic understanding of lattice defects is microscopy.21) The films generated high-density interfacial extremely insufficient. misfit-induced dislocations and related planar defects, SnO2 rutile oxide thin films are typical ionic metal oxides through misfit relaxation. The evolution of the surface mor- exhibiting unique electronic and optical properties and are phology and cross-sectional structure for thinner films sug- attractive for applications to transparent conductive ele- gested that the introduction of such defects occurs at the ctrodes, polarizing filters, gas sensors, and optical early growth stage, due to a large lattice misfit, and is catalyses.3)-7) Poly-nanocrystalline thin films are of sig strongly affected by point defect condensation. Here, we nificant interest especially for application to integrated pla report on a further detailed lattice defect structure compli- nar array gas sensors, and several detailed structural catedly formed in the heteroepitaxially grown SnO2 thin research works have been performed on crystallite size dis films, mainly focusing on planar defects, since the precise tribution and lattice defects governing the sensor analysis and control of the characteristics of planar defects response.8)-10) The nanocrystalline films reportedly includ are essential for realizing future integrated high-perfor- ed undissociated dislocations, {101} crystallographic shear mance devices. plane (CSP) defects9)'10) and {101} twins,11),12)and the CSP defects acted as sinks for nonstoichiometric composition of 2. Experimental procedures oxygen vacancies, depending on their displacement SnO2 thin films were fabricated on (100) TiO2 rutile sin- 86 Toshimasa SUZUKI et al. Journal of the Ceramic Society of Japan 110 [2] 2002 87 gle-crystal substrates by ablating pressed SnO2 powder dis ks, which are of high purity (99.99%), with an ArF excimer laser. The substrates were annealed in a furnace at 1000•Ž under oxygen atomosphere (1 atm) before deposition. Films were grown at a substrate temperature of 500•Ž and under a partial oxygen pressure of 13.3Pa. The deposition rates were adjusted to 0.3-0.5nm/min, which were relative- ly low growth rates. Crystallographic defect structures of the thin films were evaluated by transmission electron microscopy (Model 002B, Topcon, Tokyo) with a high-resolution charged-cou- pled device (CCD) camera (MegaScan, Gatan Inc., Pleasanton, CA). Thin film specimens for TEM observation were prepared by mechanical polishing and were then milled by low-incident-angle Ar ion milling (precision ion polishing system (PIPS), Gatan Inc., Pleasanton, CA). 3. Results and discussion Figures 1 and 2 show cross-sectional [010] and [001] high-resolution transmission electron microscopy (HR- TEM) images of a SnO2 thin film heteroepitaxially grown on a (100) TiO2 single crystal substrate and the insets show the corresponding selected area electron diffraction pat- Fig. 1. (010)-projected cross-sectional HR-TEM image of SnO2 terns. The selected-area electron diffraction (SAD) pat thin film heteroepitaxially grown on a (100) TiO2 substrate. The in terns, each composed of only two patterns of the rutile set shows a corresponding selected-area electron diffraction pat- structure, SnO2 film and TiO2 substrate, exhibit a well-de- tern. fined feature of heteroepitaxial growth with a crystallo- graphic relationship of [010] (100)SnO2//[010](100)TiO2, despite a largely lattice-mismatched system of SnO2 thin film and TiO2 substrate, where the lattice mismatches of lat- tice parameters a and c are approximately 3 and 8% at a growth temperature of 500•Ž, respectively (unconstrained lattice parameters of SnO2 and TiO2:aSnO2=bSnO2=0.475nm nO2=0.320nm, aTiO2=bTiO2=0.461nm,, CS and CTiO,=0.297nm at 500•Ž). In both diffractions, the inner diffraction spots corresponding to an epitaxially grown SnO2 film are notably diffused in comparison to the outer sharp diffraction spots of the TiO2 substrate, suggesting a significant deterioration in crystallinity probably due to misfit relaxation. In fact, the calculated out-of-plane lattice parameter of the a-axis was a =0 .4751nm, and in-plane lattice parameters of c- and b-ax- es of SnO2 film were b=0.4735nm and c=0.3154nm, in- dicating that the biaxial compressive misfit strain of the film is almost fully relaxed in both in-plane directions. In the (010) projection of cross-sectional HR-TEM, high-density interfacial dislocation cores seemingly related to such misfit relaxation and inclined (101) planar defects originating in the plane of the SnO2/TiO2 interface are clearly visible. Some of the planar defects extend through to the uppermost surface, while the others are terminated in the film, oc- casionally forming overlaid wedge-shaped defects. From the Fig. 2. (001)-projected cross-sectional HR-TEM image of SnO2 closure failure of their Burgers circuits, the Burgers vector thin film heteroepitaxially grown on a (100) TiO2 substrate. The in set shows a corresponding selected-area electron diffraction pat- components of the interfacial dislocations projected on the tern. micrograph of the (010) plane are classified into two types, 1/2[101] of partial type and [001] of complete type. In the (001) projection, the high-density misfit-induced interfacial
Details
-
File Typepdf
-
Upload Time-
-
Content LanguagesEnglish
-
Upload UserAnonymous/Not logged-in
-
File Pages6 Page
-
File Size-