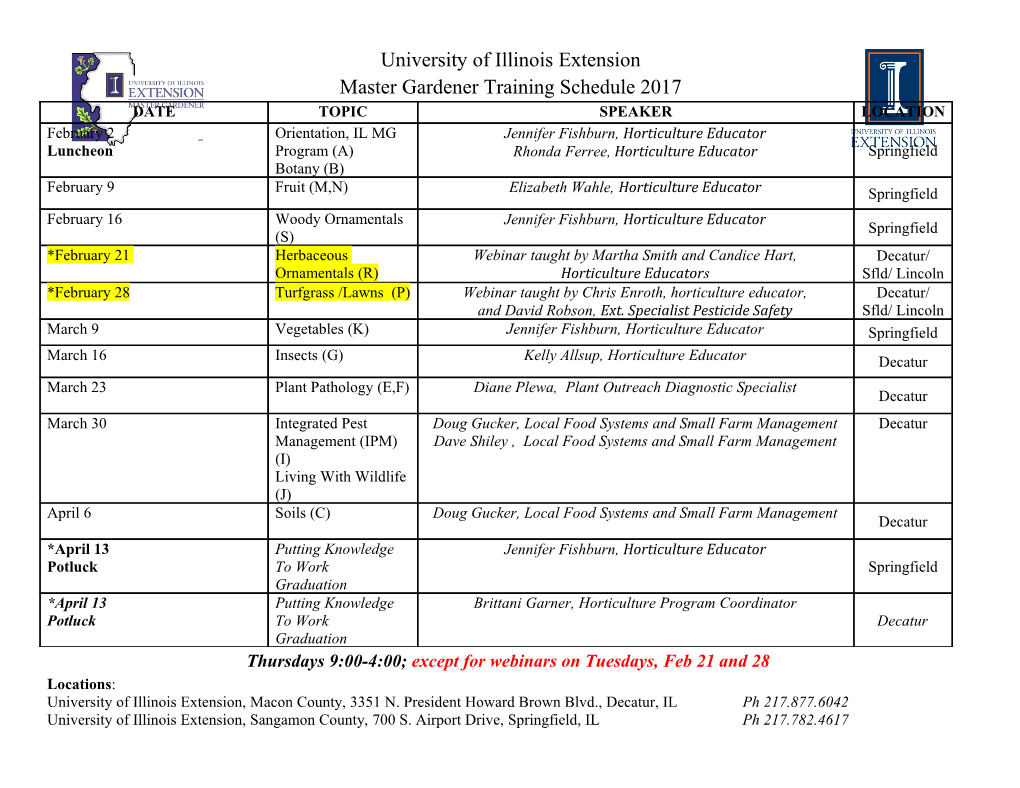
Accepted Manuscript Fault deformation, seismic amplitude and unsupervised fault facies analysis: Snøhvit Field, Barents Sea Jennifer Cunningham, Nestor Cardozo, Christopher Townsend, David Iacopini, Gard Ole Wærum PII: S0191-8141(18)30328-6 DOI: 10.1016/j.jsg.2018.10.010 Reference: SG 3761 To appear in: Journal of Structural Geology Received Date: 10 June 2018 Revised Date: 11 October 2018 Accepted Date: 11 October 2018 Please cite this article as: Cunningham, J., Cardozo, N., Townsend, C., Iacopini, D., Wærum, G.O., Fault deformation, seismic amplitude and unsupervised fault facies analysis: Snøhvit Field, Barents Sea, Journal of Structural Geology (2018), doi: https://doi.org/10.1016/j.jsg.2018.10.010. This is a PDF file of an unedited manuscript that has been accepted for publication. As a service to our customers we are providing this early version of the manuscript. The manuscript will undergo copyediting, typesetting, and review of the resulting proof before it is published in its final form. Please note that during the production process errors may be discovered which could affect the content, and all legal disclaimers that apply to the journal pertain. ACCEPTED MANUSCRIPT 1 Fault deformation, seismic amplitude and unsupervised fault facies 2 analysis: Snøhvit field, Barents Sea 3 Jennifer CUNNINGHAM * A, Nestor CARDOZO A, Christopher TOWNSEND A, David 4 IACOPINI B and Gard Ole WÆRUM C 5 A Department of Energy Resources, University of Stavanger, 4036 Stavanger, Norway 6 *[email protected] , +47 461 84 478 7 [email protected] , [email protected] 8 9 BGeology and Petroleum Geology, School of Geosciences, University of Aberdeen, Meston 10 Building, AB24 FX, UK 11 [email protected] 12 C Equinor ASA, Margrete Jørgensens Veg 4, 9406 Harstad, Norway 13 [email protected] 14 15 Keywords: faults, dip distortion, seismic attributes, seismic amplitude, fault facies. ACCEPTED 1 ACCEPTED MANUSCRIPT 16 Abstract 17 We present an integrated seismic imaging and fault interpretation workflow to characterize 18 the seismic expression in and around an E-W trending central fault system imaged in near- 19 angle stack seismic data of the Snøhvit Field, Barents Sea. Three E-W normal fault systems 20 offset five Triassic-Lower Cretaceous seismic horizons across the field. Fault throw is largest 21 at depth and decreases with shallowing. Dip distortion (DD) decreases in magnitude and 22 extent with shallowing. Fault enhancement (FE), a filter used to detect edges, was applied on 23 a blend of tensor, semblance and dip attributes, and allowed us to classify fault zones into 24 four unsupervised seismic fault facies (mid-high FE). High FE facies occur at the center of 25 the fault zones and are abundant in the highest thrown eastern part of the field. The FE facies 26 decrease radially outwards field wide. Facies correlate with throw and dip separation 27 gradient, which are in turn related to mechanical stratigraphy controlling fault propagation. 28 We observe systematic seismic amplitude variations:MANUSCRIPT a major amplitude drop on the fault 29 plane, and a brightening and dimming linked to faul t-related synclines and anticlines, 30 respectively. Our workflow establishes a methodology for fault interpretation, linking fault 31 throw, DD, seismic attributes and fault facies classification. 32 33 1. Introduction 34 Outcrop studies ACCEPTEDprovide a large amount of information pertaining to fault displacement, 35 structure, and the chemical and physical processes involved in the formation and growth of 36 faults on both large and small scales (e.g. Barnett et al., 1987; Childs et al., 1995, 2009; 37 Eichhubl et al., 2005). However, in a subsurface environment, seismic and well data don’t 38 always give the necessary information about faults, and outcrop data are far more difficult to 2 ACCEPTED MANUSCRIPT 39 extrapolate to the subsurface (i.e. Townsend et al., 1998; Aarland and Skjerven, 1998; 40 Færseth et al., 2007; Dutzer et al., 2010; Iacopini et al., 2016). In the rare chance that a well 41 intersects a fault and core is collected from the well, vast amounts of geological and 42 petrophysical information are collected from a small rock volume contained in the fault 43 (Aarland and Skjerven, 1998; Færseth et al., 2007). These core data then need extrapolation 44 across the field using seismic data. Since a well crosscutting a fault is a rarity, seismic data 45 remain the most used method of investigation of faults in the subsurface (e.g. Townsend et 46 al., 1998; Dutzer et al., 2010; Iacopini et al., 2016). In comparison to well data, seismic data 47 provide a broader 3D understanding of faults, their displacement, linkages, and facies 48 changes both vertically and laterally. Seismic resolution, however, often presents a problem 49 when interpreting subsurface faults (Gauthier and Lake, 1993; Townsend et al., 1998; Dutzer 50 et al., 2010; Long and Imber, 2011). For typical exploration conditions (wavelet frequency = 51 30 Hz, velocity = 3000 m/s), the vertical resolution is about 25 m (¼ of the wavelength or 52 tuning thickness), and the lateral resolution after dataMANUSCRIPT migration is about 50 m (½ of the 53 wavelength or effective Fresnel zone diameter; Ashcroft, 2011). However, in the vertical a 54 bed will still be detectable well below tuning thickness (Widess, 1973). 55 Another common issue in reflection seismology when imaging faults is diffraction, which 56 occurs when a seismic wave interacts with a discontinuity (fault) or lateral heterogeneity, 57 generating a radially scattered diffracted wave (Landa and Keymar, 1998; Townsend et al., 58 1998). Seismic data, however, are standardly processed for reflections (lithological 59 boundaries) ratherACCEPTED than diffractions (faults), which are removed during data migration 60 (Landa, 2007, 2012). 61 A number of studies have aimed to characterize in detail faults in seismic data. Townsend et 62 al. (1998) was the first comprehensive analysis of small-scale faults using amplitude 3 ACCEPTED MANUSCRIPT 63 anomalies in seismic reflection data. Dutzer et al. (2010) used seismic attribute analysis to 64 study fault architecture and sealing potential. Long and Imber (2010, 2012) introduced new 65 techniques in seismic reflector dip sampling to generate maps of fault related deformation 66 (dip distortion) in a normal fault array and a relay zone. These methods focused more on 67 extracting deformation patterns from the interpreted data, rather than attributes applied 68 specifically to the seismic data. Iacopini and Butler (2011) and Iacopini et al. (2012) designed 69 a visualization workflow combining seismic attributes, opacity filtering and spectral 70 decomposition to characterize deformation surrounding thrusts in deep marine settings. 71 Iacopini et al. (2016) used crossplots of tensor, semblance and instantaneous phase attributes 72 to resolve the seismic expression of normal fault damage. They also introduced the term 73 seismic disturbance zones (complex volumes of disrupted seismic signal around faults; SDZ) 74 and proposed methods to map unsupervised seismic fault facies within these zones (Iacopini 75 et al., 2016). These facies are reconstructed through simple statistical cross plotting 76 approaches but are not directly linked to actual geMANUSCRIPTological data, such as log data or core 77 samples (Dumay and Fournier, 1988; Posamentier and Kolla, 2003; Iacopini et al., 2016). 78 An alternative approach to investigate and understand the significance of seismic geobodies 79 is through the use of forward seismic modelling (Carcione et al., 2002 and sources therein). 80 Synthetic seismic modelling has been applied to simulate the seismic expression of faults and 81 has uncovered a wealth of knowledge with respect to the seismic imaging of fault zones (e.g. 82 Botter et al., 2014, 2016, 2017a-b). These studies concluded that there is a direct correlation 83 between amplitudeACCEPTED changes across the fault and fault-related deformation, thus confirming 84 the plausibility of exploring fault zones through the use of seismic attributes (e.g. Botter et 85 al., 2014, 2016). 4 ACCEPTED MANUSCRIPT 86 The purpose of this work is to explore existing and new techniques in fault analysis to 87 investigate the geological significance of seismic geobodies enveloping faults. We also test 88 on real seismic data some of the findings from seismic modelling by Botter et al. (2014, 89 2016, 2017a-b). To accomplish these objectives, we use pre-stack depth migrated, near-angle 90 stack and depth converted 3D seismic data from the Snøhvit Field, southwest Barents Sea, 91 Norway. Our study focuses on fault-related deformation of the Upper Triassic-Lower 92 Cretaceous interval (1.5-3 km depth) using a methodology that includes data conditioning, 93 structure maps, fault throw, dip distortion, seismic attributes, seismic fault facies, and seismic 94 amplitudes. The seismically interpreted fault structure lacks well control, but it correlates 95 with structural parameters such as fault juxtaposition and dip separation gradient (strain). 96 Thus, the proposed methodology has the potential for realistic unsupervised characterization 97 of faults in the subsurface. 98 2. Geological setting MANUSCRIPT 99 The Snøhvit Field is located in the Norwegian southwest Barents Sea in the center of the 100 Hammerfest Basin (Fig. 1 a, b; Linjordet and Olsen, 1992). The Hammerfest Basin is a 101 downthrown ENE-WSW rift basin, which began to develop during the Late Jurassic- Early 102 Cretaceous (Fig. 1a; Sund et al., 1984; Berglund et al., 1986; Linjordet and Olsen, 1992; 103 Ostanin et al., 2012). The basin is 150 km long by 70 km wide and is bound to the north by 104 the Loppa High, along the southeast margin by the Finnmark Platform and to the west by the 105 Tromsø Basin (SundACCEPTED et al., 1984; Doré, 1995; Ostanin et al., 2012). The main subsidence of 106 the Hammerfest Basin was controlled by NE-SW basin bounding faults in the Early 107 Cretaceous (Fig.
Details
-
File Typepdf
-
Upload Time-
-
Content LanguagesEnglish
-
Upload UserAnonymous/Not logged-in
-
File Pages60 Page
-
File Size-