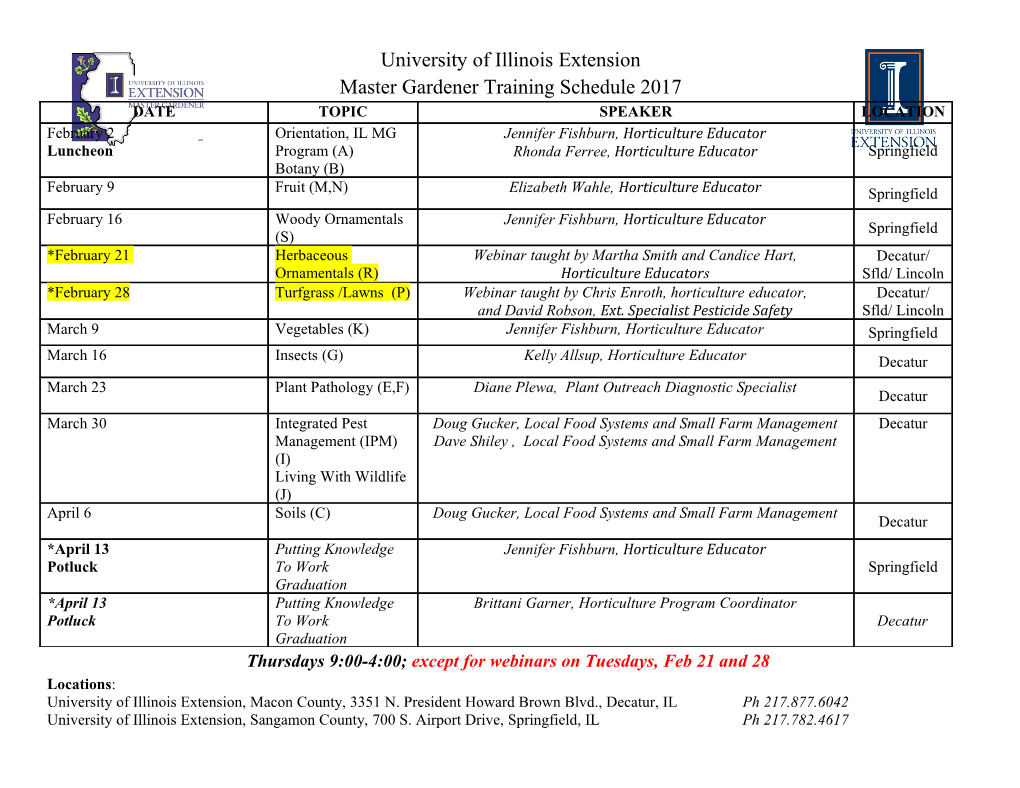
Tetrahedron: Asymmetry 17 (2006) 3021–3029 Synthesis of polymer-supported chiral lithium amide bases and application in asymmetric deprotonation of prochiral cyclic ketones Lili Ma and Paul G. Williard* Department of Chemistry, Brown University, Providence, RI 02912, USA Received 18 October 2006; accepted 9 November 2006 Abstract—Polymer-supported chiral amines were effectively prepared from amino acid derivatives and Merrifield resin. Treatment of polymer-supported amines with n-butyllithium gave the corresponding polymer-suppported chiral lithium amide bases, which were tested in the asymmetric deprotonation reactions of prochiral ketones. Trimethylsilyl enol ethers were obtained in up to 82% ee at room temperature. The polymer-supported chiral lithium amides can be readily recycled and reused without any significant loss of reactivity or selectivity. Ó 2006 Elsevier Ltd. All rights reserved. 1. Introduction CLAB’s are less likely to participate in aggregation equilib- ria. Secondly, the asymmetric deprotonation reactions More recently, chiral lithium amide bases (CLAB’s) have mediated by supported CLAB’s are possible over a large been successfully used in asymmetric reactions1 such as temperature range. Hence, it will be a great improvement the aldol reaction,2,3 alkylation,4,5 rearrangement of expox- to achieve enantioselectivity with the supported CLAB’s ides,6,7 and deprotonation of prochiral ketones.8,9 Due to at room temperature instead of the commonly used low the potential application of the chiral enolates in total syn- temperature.20 Finally, in contrast to the aggregation of thesis, asymmetric deprotonation reactions have attracted reactive species in solution, supported CLAB’s will favor special attention. For example, Simpkins et al. reported the separation of reactive species and potentially enhance the deprotonation of prochiral ketones by C2 symmetric the reactivity and enantioselectivity. Taking advantage of amides.10,11 Koga et al. synthesized diamines with different this ‘pseudodilution effect’,21 the supported CLAB’s will N-alkyl groups and subsequently applied the correspond- be significantly less dependent on additives in asymmetric ing lithium amides in the asymmetric deprotonation reac- reactions. tions.12,13 Henderson systematically studied magnesium amide base-mediated enantioselective deprotonation Herein, we report the synthesis and characterization of processes.14,15 polymer-supported CLAB’s, as well as their application in asymmetric deprotonation reactions of prochiral cyclic Despite the increasing use of polymeric chiral reagents in ketones. We also report on an optimized procedure for organic synthesis, the number of papers dealing with asym- the synthesis of polymer-supported CLAB’s with a six car- metric deprotonation using polymer-supported CLAB’s is bon spacer between the functional groups and polymer still limited.16–18 The polymer-supported CLAB’s share backbone. several common advantages as other supported reagents utilized in solid phase organic synthesis (SPOS), such as easy separation of the products, and simple recycling of supported CLAB’s by filtration, which is important for 2. Results and discussion precious demanding ligands.19 More importantly, there are three additional beneficial polymeric effects we are pur- Merrifield resin was chosen as the polymer backbone for suing with these supported CLAB’s. Firstly, the supported CLAB’s in our study because of its readily availability and the fact that the substitution of the chloromethyl group requires no harsh reaction conditions. Considering * Corresponding author. E-mail: [email protected] the total effect of the swelling ability, physical stability, 0957-4166/$ - see front matter Ó 2006 Elsevier Ltd. All rights reserved. doi:10.1016/j.tetasy.2006.11.011 3022 L. Ma, P. G. Williard / Tetrahedron: Asymmetry 17 (2006) 3021–3029 and number of reactive sites on a single bead, the com- We synthesized 4 with a six carbon spacer using a monly used chloromethylated polystyrene beads have a carbon–carbon linkage,28,29 as shown in Scheme 2. cross-linking degree between 1% and 2%, a particle size between 100 and 200 mesh, and a loading in the range of This all carbon-linker, bearing a robust carbon–carbon 1–1.5 mmol/g. The functional groups in our amide bases bond attached to the polymer backbone, requires more are readily prepared from amino acids via the correspond- synthetic steps than the alternatives discussed below. ing amino alcohols obtained according to Shioiri’s meth- Hence, after five steps, the final loading amount of amine od.22 These amino alcohols were converted into sodium is only 0.16 mmol/g. Additionally, the use of a Grignard re- alkoxides and then substituted for chloride23 on the resins agent in the first synthetic step caused difficulties in filtra- to form polymer-supported aminoethers 1a–c, as shown in tion and washing procedures. These limitations led us to Scheme 1. choose a carbon–oxygen linkage for the direct attachment of our CLAB’s to the Merrifield resin backbone. Hence, for Although these reactions are straightforward and give practical reasons, we settled on a six carbon spacer with a good to excellent yields, it was hard to attach prolinol to carbon oxygen attachment to the resin backbone,30,31 as Merrifield resin using this method. The steric hindrance shown in Scheme 3. around nitrogen is small, and hence nitrogen alkylation oc- curred much more readily than oxygen alkylation. This The procedure utilized to synthesize polymeric amines 5a could not be avoided under any reaction conditions we and 5b was modified from that reported by Johansson17 examined. Two other functional groups we incorporated and Majewski.18 The synthetic steps utilized include trans- into our polymer-supported CLAB’s were diamines synthe- halogenation, etherification, transhalogenation again and sized via a modification of Koga’s procedure.24,25 They finally amination. We found that in the presence of tetrabu- were attached to the polymer through the nitrogen atom. tylammonium iodide (TBAI), the first transhalogenation The resulting polymer-supported diamines 2a and 2b are from Cl to I is not necessary, and the etherification of Mer- also shown in Scheme 1. Polymer-supported (R)-a-meth- rifield resin takes place at room temperature with almost ylbenzylamine 3, previously reported by Henderson,16 quantitative transformation. was also examined in our study. FTIR and 13C gel phase NMR were used to characterize the structure of the func- Figure 1 lists the structure as well as the loading amount of tionalized resins, and elemental analysis exhibited the ex- polymeric amines used in our study. The maximum loading pected values for nitrogen with yields in the range of 89– amount (fmax) was calculated from the initial chloride load- 96%. ing of Merrifield resin. The conditions used to prepare polymeric lithium amides utilized excess n-BuLi at room The reactivity of bound species sometimes differs signifi- temperature for 20 min. It is important to wash off excess cantly from their unbound analogues. In general, the yields n-BuLi with a fresh solvent, otherwise butylation by-prod- decreased as a result of constrained mobility and the steric uct is observed. The amount of lithium amide on the poly- effect of the bulky polymer matrix. The constrained mobil- mer was also determined by acid–base titration of the ity makes it harder to achieve the transition state geometry methanol washings,23 and these titration values were in and the polymeric backbones slow down the diffusion of agreement with elemental analysis. reactants. This inherent limitation derived from the hetero- geneous nature of polymers can be addressed by adding a While numerous polymer-supported reagents have been spacer between the functional group and the polymer back- utilized in organic synthesis, the use of polymer-supported bone, which allows enough mobility and separates further chiral lithium amide bases is not widespread. We chose to the functional groups from the polymer backbones.26,27 study asymmetric enolization reactions assisted by poly- R R1 1 1a R1=iPr a) NaH, DMF, 4 h HO HN O N 1b R =Me Cl 1 b) , 120 oC, 2 d H 1c R1=Bn Ph Ph Cl 2a R2= N H N N c) , NaHCO3 2 N R2 H DMF, 80 oC, 2 d 2b R2= N N Cl Ph c) , NaHCO Ph 3 N H N H 3 2 DMF, 80 oC, 2 d Scheme 1. Synthesis of polymer-supported chiral amines without spacers. L. Ma, P. G. Williard / Tetrahedron: Asymmetry 17 (2006) 3021–3029 3023 Cl a) b) B c) OR' e) O HN R' =OH d) R' =OTs 4 Scheme 2. Synthesis of polymer-supported chiral amine with an all carbon spacer. Reagents and conditions: (a) ClMg(CH2)4CH@CH2,Li2CuCl4, THF, 65 °C, 2 d; (b) 9-BBN, THF, rt, 1 d; (c) Bu4NOH/MeOH, H2O2, 19 h; (d) TsCl, Et3N, DMAP, CH2Cl2, rt, 48 h; (e) sodium (S)-2-isopropylamino-3- methylbutoxide, THF, rt, 2 d. Ph a) Cl O c) O OR' N H R1 R' = OH b) R' = I 5a R1 = N 5b R1 = N N Scheme 3. Synthesis of polymer-supported chiral amines with ether spacers. Reagents and conditions: (a) HO(CH2)6OH, TBAI, NaH, DMF, rt, 2 d; (b) PPh3, imidazole, I2, THF, rt, 4 d; (c) H2NCHPhCH2R1, NaHCO3, DMF, microwave, 160 °C, 2 h. Ph O N O N O N Li Li Li Li - 1a Li - 1b Li - 1c 1.16 mmol/g 1.10 mmol/g 1.04 mmol/g fmax = 1.25 mmol/g fmax = 1.20 mmol/g fmax =1.14mmol/g Ph Ph Ph N N N N N Li Li Li N Li - 2a Li - 2b Li - 3 0.95 mmol/g 1.01 mmol/g 1.07 mmol/g fmax =1.07 mmol/g f max =1.05mmol/g fmax =1.17 mmol/g Ph Ph O N N N N N 6 6 5 Li O Li O Li N Li - 4 Li - 5a Li - 5b 0.27 mmol/g 0.45 mmol/g 0.28 mmol/g fmax =0.91mmol/g fmax =1.01mmol/g fmax =1.00mmol/g Figure 1.
Details
-
File Typepdf
-
Upload Time-
-
Content LanguagesEnglish
-
Upload UserAnonymous/Not logged-in
-
File Pages9 Page
-
File Size-