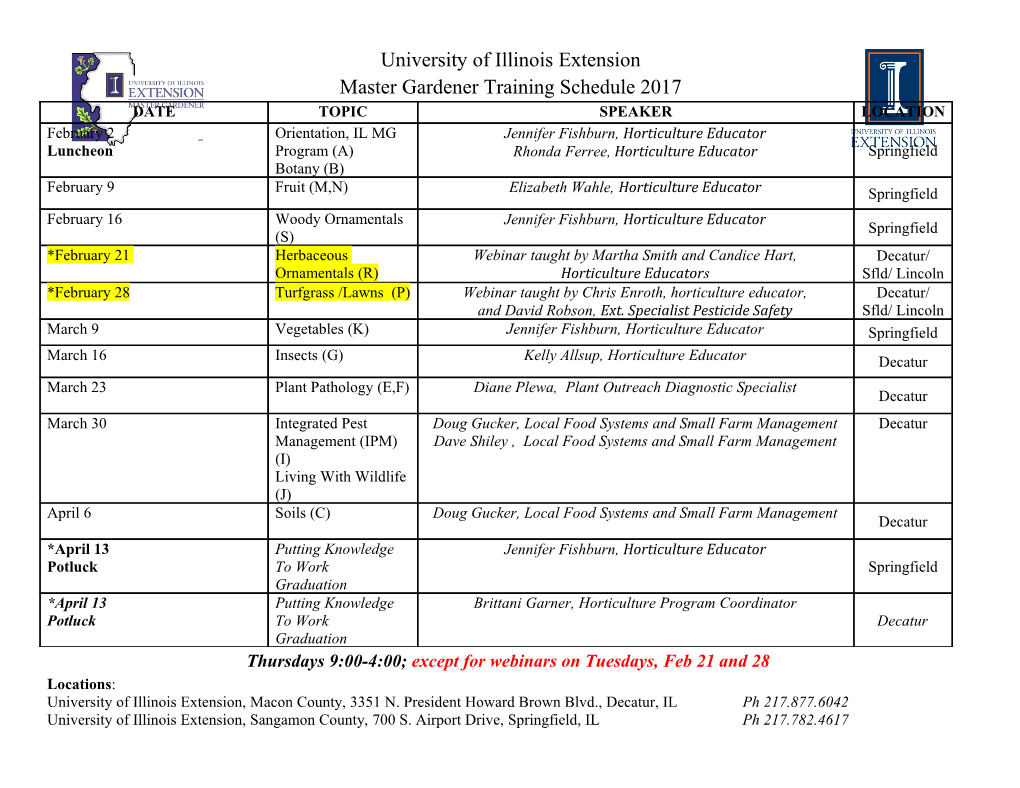
This is an open access article published under a Creative Commons Attribution (CC-BY) License, which permits unrestricted use, distribution and reproduction in any medium, provided the author and source are cited. pubs.acs.org/CR Review Electrically Conductive Metal−Organic Frameworks † † Lilia S. Xie, Grigorii Skorupskii, and Mircea Dinca*̆ Cite This: https://dx.doi.org/10.1021/acs.chemrev.9b00766 Read Online ACCESS Metrics & More Article Recommendations ABSTRACT: Metal−organic frameworks (MOFs) are intrinsically porous extended solids formed by coordination bonding between organic ligands and metal ions or clusters. High electrical conductivity is rare in MOFs, yet it allows for diverse applications in electrocatalysis, charge storage, and chemiresistive sensing, among others. In this Review, we discuss the efforts undertaken so far to achieve efficient charge transport in MOFs. We focus on four common strategies that have been harnessed toward high conductivities. In the “through-bond” approach, continuous chains of coordination bonds between the metal centers and ligands’ functional groups create charge transport pathways. In the “extended conjugation” approach, the metals and entire ligands form large delocalized systems. The “through-space” approach harnesses the π−π stacking interactions between organic moieties. The “guest-promoted” approach utilizes the inherent porosity of MOFs and host−guest interactions. Studies utilizing less defined transport pathways are also evaluated. For each approach, we give a systematic overview of the structures and transport properties of relevant materials. We consider the benefits and limitations of strategies developed thus far and provide an overview of outstanding challenges in conductive MOFs. CONTENTS 5.1. Metal-Based Hopping AA 5.2. Linker-Based Hopping AB 1. Introduction A 5.3. Mixed Metal- and Linker-Based Hopping AC 1.1. Design Strategies and Mechanisms B 6. Guest-Promoted Transport AD 1.1.1. Through-Bond Pathways D 6.1. Iodine and Polyiodides AD 1.1.2. Extended Conjugation D 6.2. Organic and Organometallic Molecules AF 1.1.3. Through-Space Pathways D 6.3. Conductive Polymers and Oxides AG 1.1.4. Redox Hopping E 7. Conclusions and Outlook AH 1.1.5. Guest-Promoted Transport E Author Information AJ 1.2. Electronic Structures of MOFs E Corresponding Author AJ 1.3. Practical Considerations E Authors AJ 2. Through-Bond Pathways G Author Contributions AJ 2.1. MOF-74-Type Frameworks G Notes AK 2.2. Azolate Frameworks H Biographies AK See https://pubs.acs.org/sharingguidelines for options on how to legitimately share published articles. 3. Extended Conjugation K Downloaded via MASSACHUSETTS INST OF TECHNOLOGY on August 11, 2020 at 15:34:02 (UTC). Acknowledgments AK 3.1. Frameworks with Ditopic Redox Noninno- Abbreviations AK cent Linkers K References AL 3.1.1. Two-Dimensional Layered Frameworks with Ditopic Linkers L 3.1.2. Three-Dimensional Frameworks Based 1. INTRODUCTION on dhbq N 3.2. Frameworks with Flat, Multitopic, Conju- Metal−organic frameworks (MOFs) are a class of porous gated Linkers N materials with high surface areas consisting of inorganic nodes 1−3 3.2.1. Semiquinoid Linker-Based Materials O connected by organic linkers. Since the inception of the 3.2.2. Thiolate Linker-Based Materials R 3.2.3. Iminosemiquinoid Linker-Based Materi- Special Issue: Porous Framework Chemistry als U 4. Through-Space Pathways W Received: November 26, 2019 4.1. Tetrathiafulvalene-Based Frameworks X 4.2. Other π−π Stacked Frameworks Z 5. Redox Hopping AA © XXXX American Chemical Society https://dx.doi.org/10.1021/acs.chemrev.9b00766 A Chem. Rev. XXXX, XXX, XXX−XXX Chemical Reviews pubs.acs.org/CR Review field in the late 1990s and early 2000s, these materials have trends, the conductivities of the materials included in this been investigated extensively for applications, such as gas Review span more than 17 orders of magnitude. − − − storage,4 7 separations,8 11 and catalysis,12 15 because of their Many other reviews pertaining to electrically conductive high porosity and chemical tunability. In contrast, the MOFs have appeared in recent years. Sun et al. first provided electronic properties of MOFs have received comparatively an overview of porous conductive MOFs in 2016,40 and little attention until recently, and the potential of this class of Medina et al. provided an update to this work.41 Several other materials as electrically conductive porous materials has only perspectives on conductive MOFs, their applications, and − begun to be realized. Despite the relative youth of this research outlooks have been written.42 46 Ko et al.,47 Zheng et al.,48 and area, many advances in designing, synthesizing, and character- Solomos et al.49 have focused on two-dimensional (2D) izing electrically conductive MOFs have been made, and the conductive MOFs, and Murase et al. analyzed mixed valency in fi fi conductive MOFs.50 Application-focused reviews have covered eld has experienced considerable expansion in just the last ve − years, the focus period for this Review (Figure 1) electrocatalysis51 and chemiresistive sensing.52 54 Stassen et al. reviewed MOF integration into electronic devices.55 Sun et al. described techniques for measuring the conductivity of MOFs.56 For a critical review of mostly nonporous conductive coordination polymers, we direct readers to the work of Givaja et al.57 1.1. Design Strategies and Mechanisms Electrical conductivity is a characteristic of a material that quantifies the efficacy of the transport of electrical charge. It is a convolution of the charge carrier concentration, n, and the carrier mobility, μ.58 Control over either of these quantities is nontrivial and requires deep understanding of the electronic structure of the material, as well as the mechanisms underlying charge transport. From a fundamental perspective, charge transport in solids can be described by one of two general mechanisms: hopping transport and ballistic (or band-like) transport (Figure 2).40 In the former, the charges transfer between discrete, nonbonded sites, where the charge carriers are localized. This mechanism Figure 1. Histogram showing cumulative number of papers focusing is common to disordered materials, glasses, and organic on aspects of electrical conductivity in MOFs from 2008 to 2019, semiconductors. In the latter, strong interactions between the grouped according to the design strategy employed. sites allow for formation of continuous energy bands with delocalized charge carriers. This mechanism is often found in crystalline inorganic materials. Electrically conductive MOFs are of considerable interest In chemical terms, an analogy can be made between these − fi from many perspectives. They are relevant as active materials two mechanisms and the Robin Day classi cation of mixed- − 59 for many applications, including electrocatalysis,16 20 chemir- valence compounds. In a material with strong covalent − − esistive sensing,21 26 and energy storage technologies.27 30 bonding, where we would expect band-like transport, the Their fundamental transport properties merit further study, as electrons are delocalized between the different statessimilar certain conductive MOFs have been predicted to host to that of a class III mixed-valence compound. In contrast, − topologically nontrivial electronic structures.31 36 Additionally, charge transport in more ionic materials is better described by they may contain arrangements of inorganic or organic the hopping mechanism. In these cases, distinct sites with moieties that are unprecedented in other materials, potentially differing valences exist, and additional energy has to be applied giving rise to new physical phenomena.37,38 They also present to transfer the electron between them−similar to a class II exciting new avenues for creativity in ligand design, self- mixed-valence compound. (Insulating materials with very low assembly, and crystal engineering. conductivities are analogous to class I mixed-valence This Review covers recent advances in the chemistry of this compounds, in which the valences are trapped and even class of materials, with emphasis on design principles, hopping is inaccessible.) experimental materials discovery, and characterization of The key difference in observable parameters for the two transport properties. We focus the majority of our discussion mechanisms lies in the temperature dependence of con- on crystalline coordination networks with potential voids39 ductivity. Hopping-type conductivity is always thermally with two- and three-dimensional connectivities for which activated: higher temperatures lead to higher conductivities. electrical conductivity values are reported. Where appropriate, This dependence follows an exponential law and is described σ σ − 1/d σ we also review chemically related materials (as demonstrated by = 0 exp[ (T0/T) ], where is the conductivity, T is σ fi by compositional and structural characterization) that do not the temperature, T0 and 0 are constants speci c to the exhibit permanent porosity. While it is important to under- material, and d is the dimensionality of the sample.60,61 stand the mechanisms underlying the transport properties of In contrast to hopping, band-like transport can be both the most conductive MOFs, we believe it can be equally thermally activated and deactivated. In metallic materials with instructive to examine structure−property relationships among sufficiently high carrier concentrations, the conductivity is materials that may not exhibit record conductivity values. In an
Details
-
File Typepdf
-
Upload Time-
-
Content LanguagesEnglish
-
Upload UserAnonymous/Not logged-in
-
File Pages45 Page
-
File Size-