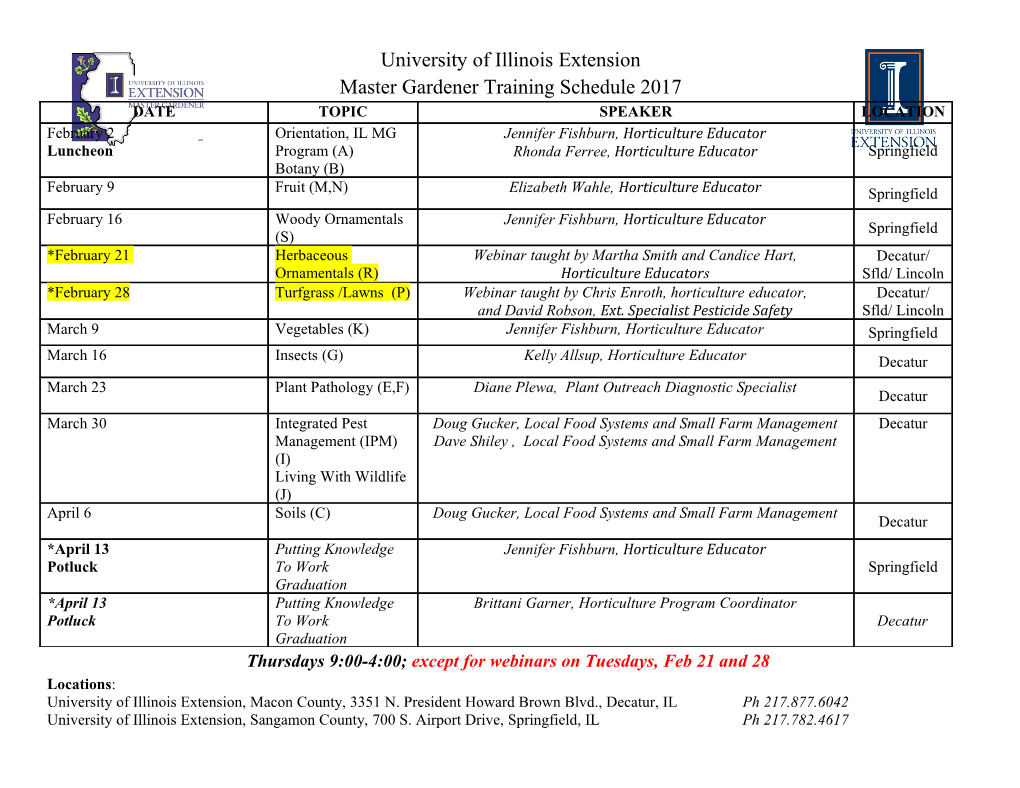
Mechanistic Studies of the Class I Ribonucleotide Reductase from Escherichia coli by Erin Jelena Artin Submitted to the Department of Chemistry in partial fulfillment of the requirements for the degree of Doctor of Science in Biochemistry at the MASSACHUSETTS INSTITUTE OF TECHNOLOGY June 2006 c Massachusetts Institute of Technology 2006. All rights reserved. Author.................................................................... Department of Chemistry May 15, 2006 Certified by . Daniel S. Kemp Professor of Chemistry Accepted by............................................................... Robert W. Field Chairman, Department Committee on Graduate Students This doctoral thesis has been examined by a committee of the Department of Chemistry as follows: Professor Daniel S. Kemp . Committee Chair Professor Catherine L. Drennan. Professor Robert G. Griffin . 2 Mechanistic Studies of the Class I Ribonucleotide Reductase from Escherichia coli by Erin Jelena Artin Submitted to the Department of Chemistry on May 15, 2006, in partial fulfillment of the requirements for the degree of Doctor of Science in Biochemistry Abstract Ribonucleotide reductases (RNRs) catalyze the conversion of nucleotides to deoxynucleotides, providing the monomeric precursors required for DNA replication and repair. The class I RNRs are found in many bacteria, DNA viruses, and all eukaryotes including humans, and are composed of two homodimeric subunits: R1 and R2. RNR from Escherichia coli (E. coli) serves as the prototype of this class. R1 has the active site where nucleotide reduc- tion occurs, and R2 contains the diferric-tyrosyl radical (Y · ) cofactor essential for radical initiation on R1. The rate-determining step in E. coli RNR has recently been shown to be a physical step prior to generation of the putative thiyl radical (S · ) on C439. Thus, the chemistry of nucleotide reduction is kinetically invisible, which has precluded detection of intermediates in the reduction process with the normal substrate. Perturbation of the system using mechanism-based inhibitors and site-directed mutants of R1 and R2 has provided the bulk of the insight into the reduction mechanism by inference. The work described in this thesis makes use of two mechanism-based inhibitors, 20 - azido - 0 0 0 0 0 0 2 - deoxyuridine - 5 - diphosphate (N3UDP) and 2 - deoxy - 2 ,2 - difluorocytidine - 5 - diphos- phate (dFdCDP), and one active site mutant, E441Q R1, to further our understanding of the catalytic capabilities of RNR. The results provide strong support for a 30 - ketodeoxynu- cleotide intermediate postulated to lie on the normal reduction pathway, as well as for the elimination of nitrogenous base in the active site of R1 during inhibition. The studies further show that under physiologically relevant reducing conditions, inhibition of RNR by the clini- cally important nucleotide analog dFdCDP is a result of covalent modification. An essential part of these studies was the development of a robust, high-yielding enzymatic method for the selective 50 - phosphorylation of cytidine, 20 - deoxycytidine, 20 - deoxyuridine and their analogs that are not amenable to standard chemical phosphorylation methods. Thesis Supervisor: JoAnne S. Stubbe Title: Novartis Professor of Chemistry and Professor of Biology 3 4 Acknowledgments I am grateful to Isaac Colbert and Toni Robinson for the help, support and encouragement they have given me, and for their personal integrity. Professors Catherine Drennan, Sylvia Ceyer, and Stuart Licht guided me through the graduation process within the Chemistry Department. I thank the members of my thesis committee, Professors Catherine Drennan, Robert Griffin, and Daniel Kemp for their advice, care, and time. Many of the accomplishments described in this thesis would not have been possible without the involvement of colleagues and collaborators. John Robblee, Jie Ge, Deborah Perlstein, Debora Martino, Galit Bar, Nicholas Lees, Gregory Lohman, Mohammad Seyed- sayamdost, Aaron Hoskins, Stanislaw Wnuk, J¨org Fritscher, Yongting Wang, and Bernd Giese were always ready to share their expertise and provide encouragement. I thank them for helping me become a better scientist. The manuscript itself has been immeasurably improved by Christopher Wipf’s insightful comments, suggestions on style, and his knowledge of English grammar. When I grow up, I hope to be able to write like he. Helpful comments on parts of this manuscript were supplied by friends and colleagues. I am most grateful to them all: John Robblee, Debora Martino, Danil Suits, and Jun Wang. I owe a special debt to John Robblee for his technical input on Chapters 2, 4, and 5. Completion of this manuscript would not have been possible without Ben Artin’s con- tribution to the figures and formatting. That I felt that I could rely on him with complete confidence was a gift for which I am grateful. Professor Catherine Drennan spent many hours proofreading this manuscript and steering it through many stages of completion. Ben Artin, Christopher Wipf, and Tijana Antoni´cadvised me, inspired me, encouraged me, and sustained me. Most of all, they believed in me at times when I didn’t think it was at all clear that confidence was warranted. This thesis is dedicated to Budimka and Tripko Mili´cevi´c, my grandparents, who intro- duced me to the joy of understanding the world, with gratitude, admiration, and love. 5 6 Contents 1 Introduction 17 1.1 General background . 17 1.2 Structure . 18 1.3 Kinetic model for E. coli RNR . 20 1.4 Proposed mechanism of ribonucleotide reduction . 21 1.5 Mechanism-based inhibition of RNR . 22 1.6 Evidence for the proposed mechanism of ribonucleotide reduction . 23 1.7 Scope of this thesis . 23 Bibliography . 25 2 Structure of the nitrogen-centered radical formed during inactivation of Class I Escherichia coli ribonucleotide reductase by 20 - azido - 20 - de- oxyuridine - 50 - diphosphate: trapping of the 30 - ketonucleotide 29 2.1 Introduction . 29 2.2 Materials and methods . 31 2.2.1 Purchased materials and materials obtained as gifts . 31 2.2.2 Protein overexpression, purification, and activity assays . 31 2.2.3 Examination of the reaction of N3UDP and RNR by 9 GHz EPR spec- troscopy . 32 2.2.4 Examination of the reaction of N3UDP and RNR by 140 GHz EPR spectroscopy . 32 2.2.5 Simulations of the EPR spectra . 33 2.2.6 Computational methods . 33 2.3 Results . 33 2.3.1 9 GHz EPR experiments . 34 2.3.2 140 GHz EPR experiments . 35 2.3.3 Quantum chemical calculations to assign the structure of N · . 36 2.4 Discussion . 40 2.4.1 Structural assignment of N · ....................... 40 2.4.2 Mechanistic implications . 42 2.5 Acknowledgments . 44 Bibliography . 44 7 8 Contents 3 Development of a high-yielding enzymatic method for the 50-phosphorylation of C, dC, dU and their 20-analogs 49 3.1 Introduction . 49 3.2 Materials and methods . 52 3.2.1 Purchased materials and materials obtained as gifts . 52 3.2.2 Growth and induction of 6xHis - HdCK expression strain . 52 3.2.3 Purification of 6xHis - HdCK . 53 3.2.4 Spectrophotometric and radioactive assays of 6xHis - HdCK . 54 3.2.5 Growth and induction of human GST - UMP - CMP kinase . 54 3.2.6 Purification of human GST - UMP - CMP kinase . 55 3.2.7 Assay of human GST - UMP - CMP kinase . 55 3.2.8 Formation of nucleoside monophosphates . 55 3.2.9 Formation of nucleoside diphosphates . 56 3.2.10 Purification of dCDP, dUDP, and their 20-analogs after enzymatic diphosphorylation . 56 3.2.11 Purification of CDP after enzymatic diphosphorylation . 57 3.3 Results . 57 3.3.1 Growth and induction of 6xHis - HdCK expression strain . 57 3.3.2 Purification and activity assays of 6xHis - HdCK . 58 3.3.3 Growth and induction of human GST - UMP - CMP kinase . 60 3.3.4 Purification and activity assays of human GST - UMP - CMP kinase . 60 3.3.5 Formation of nucleoside monophosphates . 61 3.3.6 Formation of nucleoside diphosphates . 62 3.3.7 Purification of dCDP, dUDP, and their 20-analogs after enzymatic diphosphorylation . 62 3.3.8 Purification of CDP after enzymatic diphosphorylation . 63 3.4 Discussion . 64 Bibliography . 66 4 Effect of removing the general acid/base catalyst E441 from the RNR re- action: evidence for the formation of an α,β-unsaturated 20 - ketyl radical anion 69 4.1 Introduction . 69 4.2 Materials and methods . 73 4.2.1 Purchased materials and materials obtained as gifts . 73 4.2.2 Protein overexpression, purification, and activity assays . 73 4.2.3 Tyrosyl radical loss on R2 . 76 4.2.4 Synthesis of 5,6 - dideuteriocytidine . 76 4.2.5 Synthesis of [20 - 2H] - cytidine . 77 4.2.6 Enzymatic phosphorylation of specifically deuterated cytidine analogs 77 4.2.7 9 GHz EPR experiments and simulations . 79 4.2.8 140 EPR experiments . 80 Contents 9 4.2.9 35 GHz ENDOR experiments . 81 4.3 Results . 82 4.4 Discussion . 94 4.5 Acknowledgments . 97 Bibliography . 97 5 Inactivation of aerobic Escherichia coli ribonucleotide reductase by 20,20 - difluoro - 20 - deoxycytidine - 50 - diphosphate: studies on the structure of a substrate-based radical and evidence for covalent modification 101 5.1 Introduction . 101 5.2 Materials and methods . 103 5.2.1 Purchased materials and materials obtained as gifts . 103 5.2.2 Protein overexpression, purification, and activity assays . 104 5.2.3 Time-dependent inactivation of R1 and R2 by dFdCDP in the presence and absence of reductants . 108 5.2.4 Tyrosyl radical loss on R2 in the presence of dFdCDP . 109 5.2.5 Phosphorylation of [5 - 3H] - dFdC to form [5 - 3H] - dFdCMP . 109 5.2.6 Phosphorylation of [5 - 3H] - dFdCMP to form [5 - 3H] - dFdCDP . 109 5.2.7 Phosphorylation of [10 - 2H] - dFdC to form [10 - 2H] - dFdCMP . 110 5.2.8 Phosphorylation of [10 - 2H] - dFdCMP to form [10 - 2H] - dFdCDP . 111 5.2.9 Size-exclusion experiments with radio-labeled dFdCDP to look for ev- idence of covalent modification .
Details
-
File Typepdf
-
Upload Time-
-
Content LanguagesEnglish
-
Upload UserAnonymous/Not logged-in
-
File Pages164 Page
-
File Size-