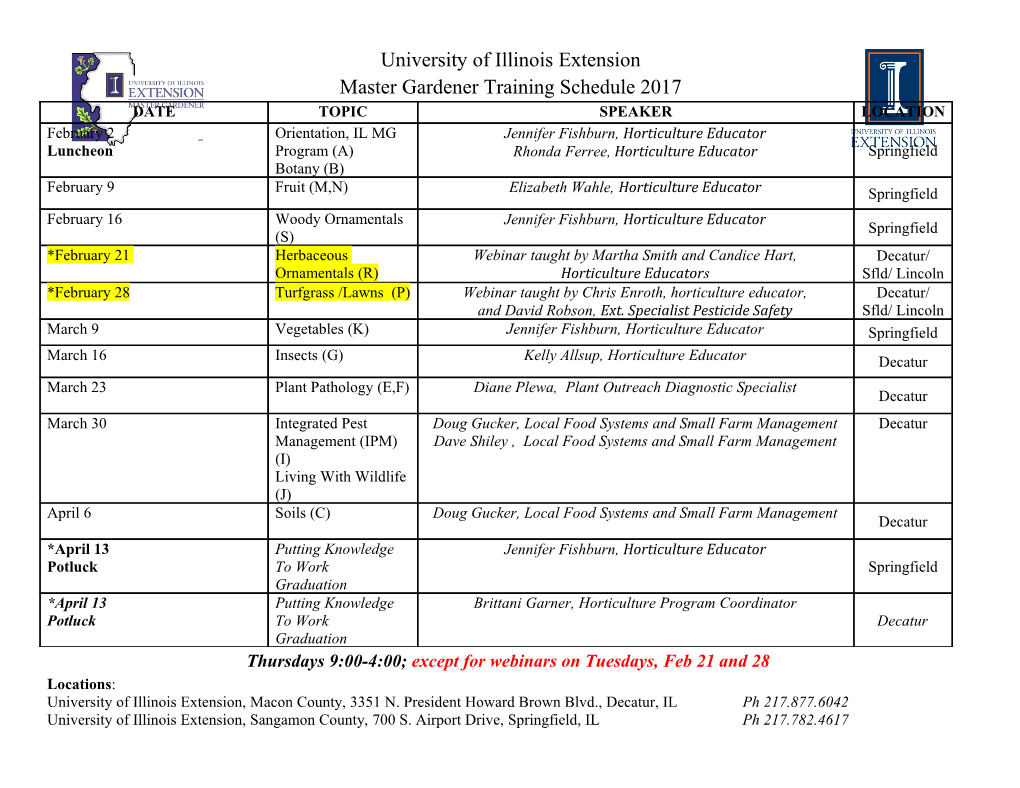
Master Thesis essay: Investigating the utility of the optogenetic toolbox for cell cycle synchronization in budding yeast 16 October 2019 Author: Mart Bartelds MSc Biomolecular Sciences Supervisor: Dr. Andreas Millias Argeitis Molecular Systems Biology group University of Groningen Abstract Cell division in eukaryotes is achieved via a conserved and tightly controlled protein network. In order to study processes that happen at specific stages during this division cycle it is important to have a culture with synchronized cells. Currently used synchronization methods often use the ‘arrest-and-release’ strategy, in which cells are arrested at a specific point in the cell cycle using chemicals or conditional mutants. Releasing the cells from the arresting conditions results in a synchronized re-entry to the cell cycle. However, these methods usually have severe side-effects on cell physiology and the switching between the restrictive and permissive state is slow. To overcome these limitations, optogenetic systems may be used, as these systems can offer exact molecular control over diverse cellular processes and switching between two states can be achieved rapidly. To identify potential targets for optogenetic control an overview is given of natural existing cell cycle arresting pathways. Two exiting optogenetic systems were identified that utilize these pathways. Since these systems were not designed for cell synchronization, ways to further improve these systems for cell synchronization were discussed. Moreover, two other pathways were identified that showed high potential for cell synchronization. Finally, two papers are discussed that developed systems for direct control of the expression or degradation of key regulators of the cell cycle. Although these systems can potentially invoke less severe side-effects, the arrest is less stringent. Overall, although most optogenetic-based systems would require more optimization to be able to compete with existing cell synchronization methods, these system have the potential to overcome the limitations of the current synchronization methods in the future. 2 Table of contents Abstract……………………………………………………………………………………………………..2 Chapter 1: Introduction………………………………………………………………………………4 Chapter 2: Cell cycle checkpoints………………………………………………………………..6 2.1 Start transition checkpoints 6 2.2 Post-START checkpoints 7 2.3 Selection of checkpoints for optogenetic manipulation 8 Chapter 3: Hijacking the natural checkpoints………………………………………………9 3.1 The mating pheromone pathway 9 3.2 DNA replication initiation 11 3.3 The morphogenesis checkpoint 13 Chapter 4: Direct control over the central cell cycle regulators…………………..15 Chapter 5: Discussion…………………………………………………………………………………17 References…………………………………………………………………………………………………19 3 Chapter 1: Introduction Key regulators of the cell division cycle: Cell division is one of the fundamental processes of life. In eukaryotes the division cycle can be separated into four stages [Figure 1]. The two growth phases, G1 and G2, mainly serve to increase the cell mass and double the amount of proteins and organelles. In between the DNA is replicated during the S-phase and in the end the cell is divided during the M-phase (Cui, 2017). It should be noted that the budding yeast cell cycle slightly deviates from this general model, since processes characteristic to the G2 are already active during the S- phase. The main regulator of the budding yeast cell cycle is a cyclin dependent kinase (Cdc28). As the name suggests, this kinase is dependent upon the association with a class of proteins, called cyclins, for its activation and to direct it towards specific targets. The expression and degradation of these cyclins is tightly regulated, in such a way that specific cyclins are present during each stage of the cell cycle (Cui, Figure 1: Overview of the yeast cell cycle Image copied from (Cui, 2017); The middle ring shows the four 2017). Together Cdc28 and the cyclins stages of the cell cycle: G1, S, G2 and M. In the outer ring form the main regulatory unit that cartoons represent the appearance of budding yeast during orchestrates the processes that are each stage. Also several cell division processes that occur at active during most of the cell cycle. characteristic points during the cell cycle are indicated. Cell cycle synchronization: Although the discovery of the key regulators of the cell cycle was awarded with the Nobel Prize in 2001, our knowledge about the precise regulatory mechanisms is still far from complete. One of the main challenges in studying the cell cycle is that events are usually happening in a specific stage of the cell cycle, while in an exponentially growing culture cells are present in all stages. To study the processes during a specific stage the cell cycle it can be synchronized in a cell culture. A synchronized cell culture can be obtained by separating the cells in a specific stage from those in other stages or by arresting all cells at a specific stage in the division cycle and after lifting the arrest the cells will simultaneously re-enter the cell cycle. The separation method relies on the difference in physical properties of cells in different stages of the cell cycle, for example cells can be separated based on their size by centrifugal elutriation (Banfalvi, 2008). For the arrest and release method either chemicals or conditional mutants are used to arrest the cells. Examples of commonly used chemicals are mating pheromone, hydroxyurea and nocodazole (Banfalvi, 2017) of which the mechanisms of action will be discussed later on. Many temperature cell division cycle (cdc) mutants have been discovered during the Seventies in an attempt of finding genes involved in the cell 4 cycle. These mutants are functional when the cells are grown above a certain permissive temperature, but lose their function when the temperature is lowered resulting in an arrest of the cell cycle. (L. H. Hartwell, Culotti, & Reid, 1970; Leland H Hartwell, Mortimer, Culotti, & Culotti, 1973). Although all of the methods described above have specific advantages and disadvantages, they usually have severe side-effects on cell physiology and the release or synchronization process is not very fast. Optogenetic systems for cell synchronization: To tackle these two problems in cell cycle synchronization the optogenetic toolbox will be explored in this literature review. First of all, light can be instantly removed from a cell culture by simply switching the light off, which allows for a fast release. Secondly, optogenetic systems have been described that control precise molecular interactions without interfering with the general cell physiology. So far, optogenetic systems have been described to control gene expression, protein localization, degradation and dimerization (Repina, Rosenbloom, Mukherjee, Schaffer, & Kane, 2017). Here all of these methods will be encountered during the discussion of exiting and potentially new systems for the optogenetic synchronization of the budding yeast cell cycle. To achieve this goal two strategies can be discriminated. The first approach aims to activate the naturally existing cell cycle checkpoints. These checkpoints are signalling pathways that cause a delay or arrest of the cell cycle upon activation. Light inducible cell cycle arrest could be achieved by putting key interactions from these pathways under optogenetic control. The other strategy relies on directly controlling the expression or degradation of the key regulators of the cell cycle, such as for example the cyclins. In the next chapter an overview of the most important checkpoints will be given of which the most promising will be selected. In chapter 3 the selected pathways will be discussed in more detail and a strategy will be proposed to put them under optogenetic control. Next, the potential of putting the key regulators under direct optogenetic control will be discussed based on two existing systems. Finally, the results will be summarized and other potential uses for optogenetic systems in cell cycle research will be shortly discussed. 5 Chapter 2: Cell cycle checkpoints 2.1 Start transition checkpoints: Cell cycle initiation: Under constant conditions, exponentially growing yeast cells will enter the cell cycle when they have reached a certain critical mass. The exact mechanisms by which cells control their size remain a topic of discussion, which is left out of the scope of this review (Jorgensen & Tyers, 2004). Eventually, cell growth leads to the accumulation of the initial cyclin, Cln3, allowing it to activate Cdc28. The activated Cdc28/Cln3 complex triggers a positive feedback loop involving Cln1,2 and Whi5, which is well understood (Skotheim, Di Talia, Siggia, & Cross, 2008). In fact a model has been developed to simulate the START transition and it is able to capture the effect of perturbations of the central components quite well (Adames et al., 2015; Chen et al., 2004; Laomettachit, Chen, Baumann, & Tyson, 2016). Since triggering this transition irreversibly commits cells to a division cycle, there are several checkpoint pathways that can inhibit the activation of Cdc28. Metabolism affects initiation timing: To begin the availability of enough nutrients is essential for progression through the cell cycle. Nutrient depletion is mainly sensed through the PKA and Tor pathways and will lead to prevention of START. However, these pathways are also intertwined in regulation of the metabolism, which in turn modulates START as well. Furthermore, when nutritional depletion
Details
-
File Typepdf
-
Upload Time-
-
Content LanguagesEnglish
-
Upload UserAnonymous/Not logged-in
-
File Pages23 Page
-
File Size-