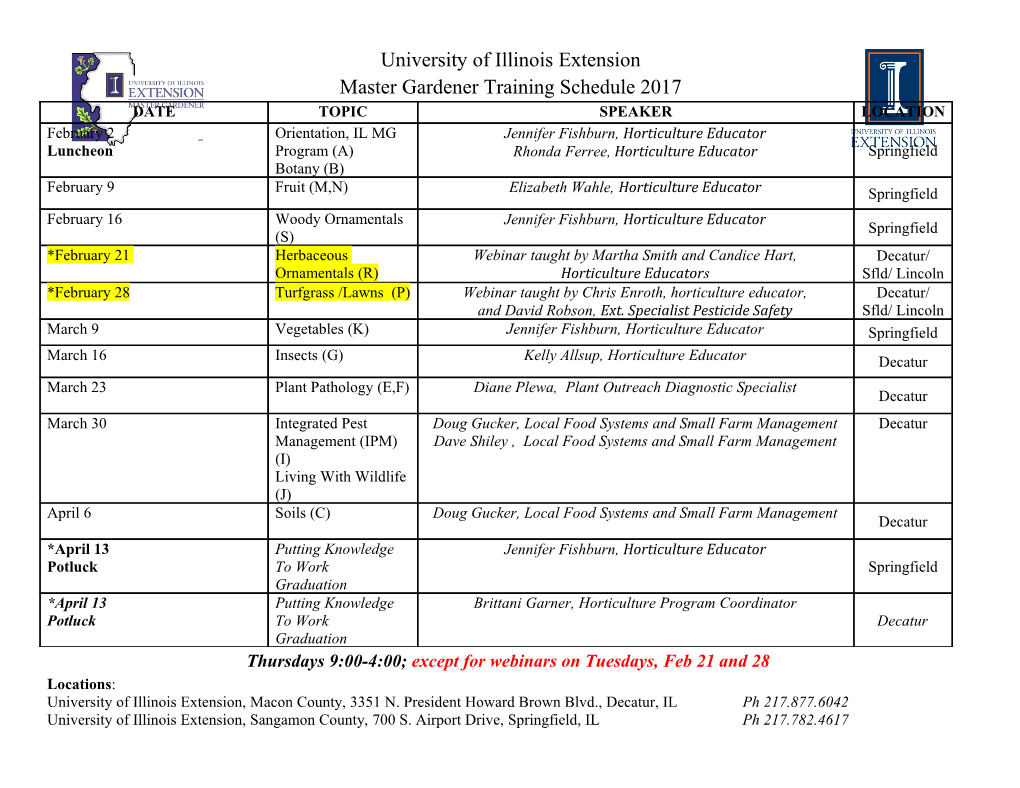
Noname manuscript No. (will be inserted by the editor) Magnetic Field Generation in Stars Lilia Ferrario · Andrew Melatos · Jonathan Zrake Received: date / Accepted: date Abstract Enormous progress has been made on observing stellar magnetism in stars from the main sequence (particularly thanks to the MiMeS, MAGORI and BOB surveys) through to compact objects. Recent data have thrown into sharper relief the vexed question of the origin of stellar magnetic fields, which remains one of the main unanswered questions in astrophysics. In this chapter we review recent work in this area of research. In particular, we look at the fossil field hypothesis which links magnetism in compact stars to magnetism in main sequence and pre-main sequence stars and we consider why its feasibility has now been questioned particularly in the context of highly magnetic white dwarfs. We also review the fossil versus dynamo debate in the context of neutron stars and the roles played by key physical processes such as buoyancy, helicity, and superfluid turbulence, in the generation and stability of neutron star fields. Independent information on the internal magnetic field of neutron stars will come from future gravitational wave detections. Coherent searches for the Crab pulsar with the Laser Interferometer Gravitational Wave Observatory (LIGO) have already constrained its gravitational wave luminosity to be . 2% of the observed spin-down luminosity, thus placing a limit of . 1016 G on the internal field. Indirect spin-down limits inferred from recycled pulsars also L. Ferrario Mathematical Sciences Institute, The Australian National University, ACT2601, Australia E-mail: [email protected] Andrew Melatos School of Physics, University of Melbourne, Australia E-mail: [email protected] Jonathan Zrake arXiv:1504.08074v1 [astro-ph.SR] 30 Apr 2015 Kavli Institute of Particle Astrophysics and Cosmology, Stanford University, USA E-mail: [email protected] 2 Ferrario, Melatos, Zrake yield interesting gravitational-wave-related constraints. Thus we may be at the dawn of a new era of exciting discoveries in compact star magnetism driven by the opening of a new, non-electromagnetic observational window. We also review recent advances in the theory and computation of mag- netohydrodynamic turbulence as it applies to stellar magnetism and dynamo theory. These advances offer insight into the action of stellar dynamos as well as processes which control the diffusive magnetic flux transport in stars. Keywords Magnetic fields Main sequence stars white dwarfs neutron stars · · · 1 Introduction It was Hale (1908), one of the greatest astrophysicist of the twentieth century, who built the first spectroheliograph and used it to establish that sunspots are magnetic and grouped in pairs of opposite polarities. Further pioneering work on solar magnetism conducted by Hale and collaborators in 1919 revealed an East-West direction of polarity in the sunspots’ magnetic fields exhibiting a mirror symmetry with respect to the solar equator. Such polarity was observed to undergo inversion according to the 11 years solar cycle. This is commonly known as the “Hale-Nicholson law”. The first detection of a magnetic field in a star other than our own Sun, 78 Vir, was obtained in 1947 by Babcock. In 1958, Babcock published the first catalogue of magnetic stars and in 1960 he discovered what is still today the most magnetic main sequence star known, HD215441 ( 3.4 104 G). This became known as “Babcock’s star”. Surface fields discovered∼ × in more recent years that rival in strength that of Babcock’s star are those of HD 154708 ( 2.45 104 G; Hubrig et al. 2005), HD137509 ( 2.9 104 G; Mathys 1995;∼ Kochukhov× 2006) and HD 75049 ( 3 104 G;∼ Freyhammer× et al. 2008; Elkin et al. 2010). ∼ × Following the detections of strong fields in main sequence stars, Blackett (1947) suggested that if magnetic flux is conserved, some white dwarfs should exhibit magnetic fields of up to 107 108 G. However, spectroscopic surveys aimed at detecting magnetic fields in− white dwarfs yielded negative results (Preston 1970). Kemp (1970) argued that electrons spiralling in a magnetic field would emit linearly and circularly polarised radiation that should be detectable in the continuum of strongly magnetic white dwarfs. A spectropo- larimetric survey of white dwarfs led to the discovery of strong circular po- larisation in the continuum of the white dwarf Grw+70◦8247 (Kemp et al. 1970). Baade and Zwicky (1934) first suggested that some stars could be made up of neutrons and that a supernova could be the result of a rapid transition of a normal star into a neutron star. Baade (1942) and Minkowski (1942) found unusual emission arising from the central parts of the Crab Nebula. Later on radio pulsations in this nebula were discovered by Staelin and Reifenstein Magnetic Field Generation in Stars 3 (1968). Such radio emission had been predicted by Shklovsky (1953) as caused by relativistic electrons spiralling around magnetic field lines. Woltjer (1964) first proposed that under magnetic flux conservation, if a star contracts to the density expected in a neutron star, then the surface field strength could be amplified to values of up to 1014 1016 G. The first pulsar, a highly magnetised rapidly spinning neutron star (Pacini− 1968), was discovered in 1967 by Jocelyn Bell (Hewish et al. 1968). In this chapter we review progress made on the origin of magnetic fields in stars. The origin of magnetic fields is still a major unresolved problem in astrophysics. The “fossil field” hypothesis is often invoked to link magnetism in compact stars to magnetism on the main sequence (see Sect.3.1 and Sect. 4.1). In the fossil scenario, some fraction of the magnetic flux of the progenitor star is conserved during the collapse process, because the stellar plasma is highly conducting and hence, by Faraday’s law, the magnetic field is ‘frozen in’ to the fluid (Woltjer 1964; Ruderman 1972; Braithwaite and Spruit 2004a; Ferrario and Wickramasinghe 2006a, 2007, 2008a). Under these circumstances, the field strength B scales with radius R of the star as B R−2. A main ∝ sequence B star with radius 5R⊙ and field 1, 000G compresses to give a neutron star with a field of 1014 G; a main∼ sequence A star with radius ∼ 1.6R⊙ and field 1, 000 G compresses to give a magnetic white dwarf with a field of 107 G.∼ The fossil field scenario is indeed quite attractive and can explain the∼ existence of magnetic fields even in the strongest magnets in the universe, the so-called “magnetars”. However, the feasibility of the fossil field hypothesis does have its problems and has been recently questioned, in the context of highly magnetic white dwarfs, on the basis of recent observational results related to their binarity (see Sect. 3.2). The fossil versus dynamo- generated fields debate for neutron stars is analysed in Sect. 4.1.1. Alternative explanations for the origin of fields in stars are presented in Sect. 2, 3.2 and 4.2. In this chapter we also review recent progress in magnetohydrodynamics (MHD) turbulence theory and computation as it applies to stellar magnetism (see Sect. 5), which underpins the operation of stellar dynamos, and controls diffusive magnetic flux transport in stars. 2 Magnetism in non-degenerate stars Magnetic fields in main sequence stars have been measured mainly via spec- tropolarimetry. Different techniques measure different field properties, e.g., line-of-sight component, volume-averaged field strength, or dipole moment. A thorough review on magnetic field measurements of non-degenerate stars across the Hertzprung-Russell diagram can be found in this book in the chap- ter by Linsky & Sch¨oller. Direct measurements of magnetic fields in the chemically peculiar main sequence Ap and Bp stars, which form about 10% of stars in the 1.6 5 M⊙ range, have revealed the existence of strong ( 3 102 3 104 G) large− scale ∼ × − × 4 Ferrario, Melatos, Zrake fields (e.g. Auri`ere et al. 2007; Donati and Landstreet 2009). Landstreet et al. (2007a) found that the majority of magnetic objects have an average longitu- dinal field (line of sight component of the surface field) B > 0.25 kG in every 1 M⊙ mass bin between 2M⊙ and 9M⊙ (Bagnulo et al. 2006a; Landstreet et al. 2007a). The lack of fields below 300G, value that corresponds to the strength at which the magnetic field is in equipartition with the gas pressure in the stel- lar photosphere, is not a detection threshold effect since Auri`ere et al. (2010) have set a 3σ upper limit of longitudinal fields down to 1 10G depend- ing on stellar brightness. The absence of magnetic stars below− this cutoff has been referred to as “the Ap/Bp magnetic desert”. This indicates that Ap and Bp stars are not simply the high field tail of a continuous field distribution. Landstreet et al. (2008) also find that the field strengths seem to show some decline with stellar age but the field incidence does not. Such large scale fields are observed throughout the main sequence phase and, in more recent years, have also been observed in a small number of stars on the red giant branch (Auri`ere et al. 2008). The recent detections of sub-gauss fields in Vega (Ligni`eres et al. 2009), and possibly in the Am stars Sirius A (Petit et al. 2011) and β UMa and θ Leo (Blaz`ere et al. 2014) have unveiled a new class of magnetic stars which is at the 1 gauss end of the magnetic desert, thus potentially suggesting a di- chotomy between strong and ultra-weak magnetic fields among intermediate- mass stars (Ligni`eres et al. 2014). This intriguing observational result has prompted Braithwaite and Cantiello (2013) to investigate the origin of these low fields.
Details
-
File Typepdf
-
Upload Time-
-
Content LanguagesEnglish
-
Upload UserAnonymous/Not logged-in
-
File Pages41 Page
-
File Size-