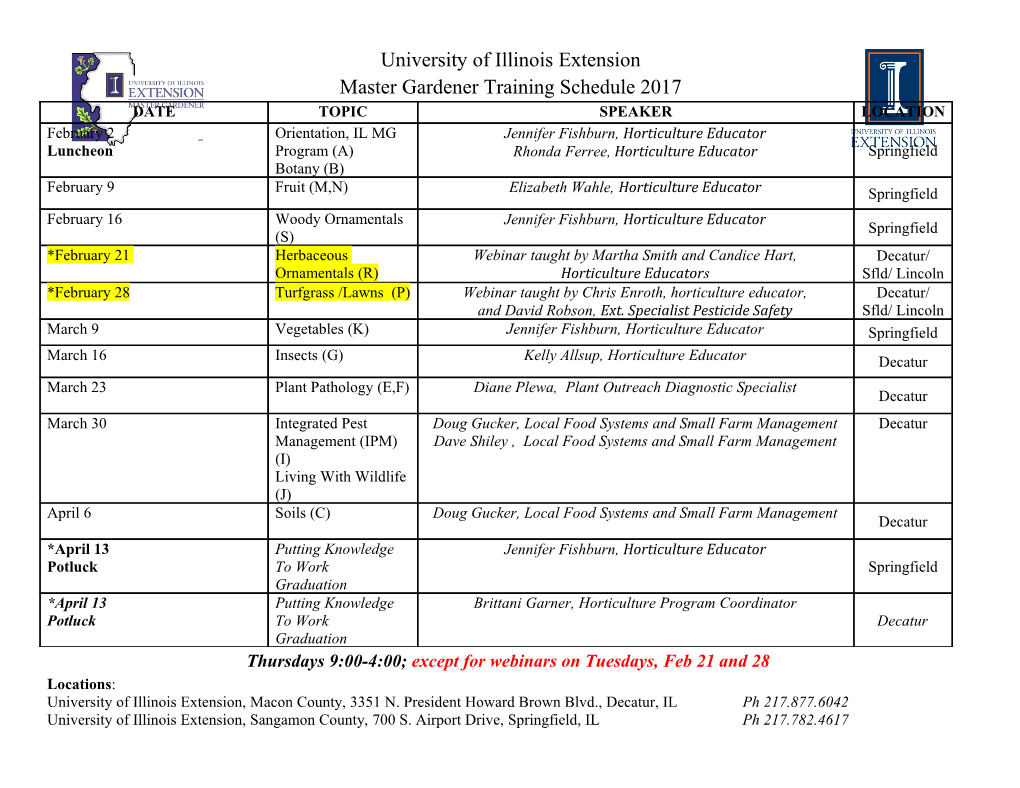
BIOSYNTHESIS OF INDIGO USING RECOMBINANT E. COLI: DEVELOPMENT OF A BIOLOGICAL SYSTEM FOR THE COST­ EFFECTIVE PRODUCTION OF A LARGE VOLUME CHEMICAL Alan Berry, Ph.D., Senior Scientist; Scott Battist, B.S., Ch.E., Development Engineer; Gopal Chotani, Ph.D., Ch.E., Senior Staff Scientist; Tim Dodge, M.S., Ch.E., Senior Scientist; Steve Peck, B.S., Ch.E., Development Engineer; Scott Power, Ph.D., Vice­ President and Research Fellow; and Walter Weyler, Ph.D., Senior Scientist Genencor International. Rochester, NY. 14618-3916. Abstract Cost-effective production of any large-volume chemical by fermentation requires extensive manipulation ofboth the production organism and the fermentation and recovery processes. We have developed a recombinant E. coli system for the production of tryptophan and several other products derived from the aromatic amino acid pathway. By linking our technology for low-cost production oftryptophan from glucose with the enzyme naphthalene dioxygenase (NOO), we have achieved an overall process for the production ofindigo dye from glucose. To successfully join these two technologies, both the tryptophan pathway and NOD were extensively modified via genetic engineering. In addition, systems were developed to remove deleterious by-products generated during the chemical oxidations leading to indigo formation. Low-cost fermentation processes were developed that utilized minimal-salts media containing glucose as the sole carbon source. Finally, economical recovery processes were used that preserved the environmental friendliness ofthe biosynthetic route to indigo. 1121 Introduction Two major problems facing the U.S. chemical industry are dependence on foreign petroleum and mounting environmental concerns and regulations (for review, see reference [1]). This has led to consideration ofbiosynthetic routes for production of aromatic compounds. Indigo dye is an example ofa large volume (worldwide production approximately 1.3 x 107 kg [1]), petroleum-derived synthetic compound for which a realistic alternative biosynthesis exists. Cost-effective biological production of indigo (or any other aromatic compound) requires that the primary raw material used be D-glucose, a feedstock that is abundant and relatively inexpensive in the U.S. This article describes the engineering ofthe aromatic amino acid pathway and ofnaphthalene dioxygenase to achieve an overall biosynthetic route from D-glucose to indigo in E. coli. The impact ofyield improvements (i.e., efficiency) in the biosynthetic process in the context ofestimated manufacturing costs are also considered. Engineering the Aromatic Amino Acid Pathway Background The conversion ofL-tryptophan to indigo in E. coli via the combined actions of the native E. coli tryptophanase and a cloned naphthalene dioxygenase (NDO) from Pseudomonas putida was first described in a landmark paper by Ensley et. al. [2]. However, indigo manufacture by simple bioconversion oftryptophan to indigo using recombinant E. coli cells is not economically viable because ofthe high cost of tryptophan. Thus, overall production of indigo from glucose via the tryptophan biosynthetic pathway represents the the only economically viable approach. Development of a tryptophan over-producing strain ofE. coli In order to overproduce tryptophan in E. coli, two key points of transcriptional regulation were removed; at DAHP synthase (the first enzyme ofthe pathway, leading to all three aromatic amino acids) and at anthranilate synthase (the first enzyme of the tryptophan branch of the pathway). We achieved this by cloning the aroG gene, encoding the DAHP synthase isozyme that is normally regulated by phenylalanine, and the entire trp operon (minus the native promoter and attenuator regions), under control of lacUV5 promoters. This removes normal transcriptional regulation ofaroG and the trp genes by phenylalanine and tryptophan, respectively, and allows controlled expression of the aroG and trp genes over a wide range using IPTG or lactose as the inducer. With respect to allosteric regulation, feedback resistant versions of all three DAHP isozymes from E. coli have been reported [3-5], and the nucleotide sequence of some of these mutant isozymes have been determined [3,4]. Likewise, mutant forms of anthranilate synthase (the trpE gene product) that are resistant to feedback inhibition by 1122 tryptophan-have been reported [6,7). In addition to being cloned under lacUV5 promoter control, the cloned aroG and trpE genes described above also contain mutations that render their products, DAHP synthase and anthranilate synthase, resistant to feedback inhibition by phenylalanine and tryptophan, respectively. The feedback resistant trpE used in this work was obtained via a random mutagenesis approach. Not suprisingly, partial sequencing of this mutant trpE gene showed that the mutation responsible for the feedback resistance is identical to one ofthe mutations (C465Y) later reported by Caligiuri and Baurle [6]. The feedback resistant aroG gene used in this work has not been sequenced. Having the approriate modified genes in hand, it is relatively straightforward to assemble a single plasmid that contains all of the genes of interest. However, plasmid copy number and stability must be considered. Stabilization of plasmids without addition of antibiotics is usually desirable for industrial strains, and several methods of achieving such stability have been described [8-10]. Integration ofcloned genes (usually w-ith a strong promoter) into the host genome has also been widely adopted. In addition to developing a plasmid construction, development of a host strain having the appropriate characteristics is equally important. Potential problems such as end product toxicity, high osmolarity and phage resistance must be considered. For tryptophan overproduction in E. coli, the only absolute requirement is that the tryptophanase (tnaA) gene be inactivated to avoid tryptophan degradation. However, we have found several other mutations in the host (both defined and undefined) to be beneficial to tryptophan production. The resulting tryptophan producing strain is grown in a fed-batch fermentation with glucose as the sole component of the feed stream. The initial glucose concentration was 1% and was fed to a final concentration of approximately 22%. A final tryptophan concentration of about 40 gIL was achieved (Fig. 1). This is well above the solubility limit oftryptophan resulting in about one-halfthe material being crystallized in the medium. For a significant portion of the tryptophan fermentation, the theoretical yield (0.227 g tryptophanlg glucose, assuming no PEP recycle [11] is achieved (Fig. 1). It has been reported that E. coli tryptophan synthase is inhibited by tryptophan at a concentration of 30 gIL leading to appearance ofindole and reduction of tryptophan production rate [12]. That did not appear to be the case with our fermentation. Similar tryptophan fermentation results to those reported here have also been achieved in Corynebacterium glutamicum [13]. Further Pathway Improvements Further improvements in tryptophan production requires manipulation of the aromatic amino acid pathway in two areas; (i) increasing the availability ofthe two precursors of aromatic biosynthesis, erythrose 4-phosphate (E4P) and PEP, and (ii) removing rate limiting steps within the common aromatic pathway. With respect to (i) above, much attention has focused on increasing the availability of PEP. Elimination or reduction of pyruvate kinase activity [14] and increasing the level 1123 ofPEP synthase activity [11] have been reported to increase intracellular levels of PEP. Furthermore, amplification ofPEP synthase has been proven to increase carbon commitment to the aromatic amino acid pathway [11]. Recently, we have found that mutants deleted for the PEP:glucose phosphotransferase system that have regained the ability to grow on glucose as sole carbon source also show an increased comittment of glucose-derived carbon to the aromatic amino acid pathway (F. Valle, N. Flores, J. Xiao and A. Berry, unpublished). This increased carbon flow has been translated into an improvement in tryptophan production in shake flask cultures (Fig. 2, left and center sets ofcolumns). The P'I'S'glucose" strain produced 2x more tryptophan than the control (PTS+) strain containing the same complement ofplasmid-borne cloned genes, and had a 2x higher specific tryptophan productivity (Qp trp). Another approach to increasing carbon commitment to the aromatic pathway in E. coli is to increase the intracellular level ofE4P. E4P is produced via the combined actions of transketolase and transaldolase.. Amplification oftransketolase (the product of the E. coli tktA gene) has been shown to have a profound positive effect on carbon commitment to the aromatic amino acid pathway [15, 16]. This increased carbon flow has also been translated into an improvement in tryptophan production in shake flask cultures (Fig. 2, right-hand set ofcolumns). Relative to the control strain, the strain containing amplified transketolase activity had a 2x increase in total carbon flow. Strangely, in this strain the carbon appeared to get restricted within the tryptophan branch ofthe pathway, resulting in a co-accumulation oftryptophan, anthranilate and indole. However, when all three compounds are added together, the effect of amplifed transketolase is similar to the results with the P'I'S'glucose" mutant (i.e., a doubling tryptophan specific productivity and concentration). In E. coli, the genes
Details
-
File Typepdf
-
Upload Time-
-
Content LanguagesEnglish
-
Upload UserAnonymous/Not logged-in
-
File Pages276 Page
-
File Size-