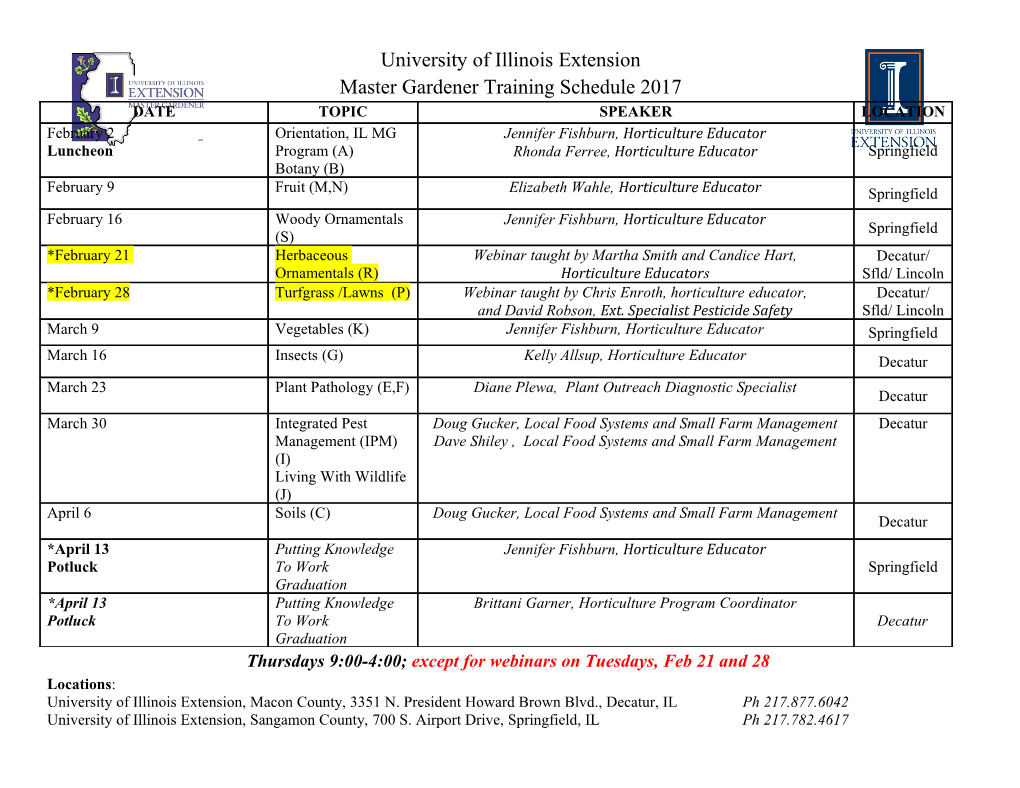
Module II: Relativity and Electrodynamics Lecture 5: Metric and higher-rank 4-tensors Amol Dighe TIFR, Mumbai Outline Metric and invariant scalar products Second rank 4-tensors: symmetric and antisymmetric Higher-rank 4-tensors Coming up... Metric and invariant scalar products Second rank 4-tensors: symmetric and antisymmetric Higher-rank 4-tensors Relating covariant and contravariant components I As observed in all examples, the change of signs of the space components of a covariant vector converts it to a contravariant one, and vice versa. The covariant and contravariant components thus contain the same information, and describe the same 4-vector. I If a 4-vector X has covariant componentsX m and contravariant componentsX k , they can be related through @x @xk = m k ; k = : Xm k X X Xm (1) @x @xm I These matrices, which “lower” or “raise” the indices of the 4-vectors are the “metrics”: @x @xk = k ; km = : gkm m g (2) @x @xm km Here in Special Relativity, gkm = g = Diag(1; −1; −1; −1). At the moment, the metrics may be just considered as matrices. Later we shall discuss their “tensorial” nature. Scalar products in terms of the metric I We saw earlier that the inner product (product with all indices summed over) of a covariant and a contravariant vector is 0m 0 k invariant under Lorentz transformation:X Ym = X Yk . k I Using Ym = gmk Y , one may write this as m k X · Y = gmk X Y (3) Thus one can talk about a “scalar product”X · Y of two vectors X and Y, without referring to their components explicitly. I The scalar product of two 4-vectors is invariant under Lorentz transformations, and hence is a frame-independent physical quantity. Proper distance in special relativity 2 m m k s = x xm = gmk x x (4) is the square of the “length” of the 4-vector x. The “proper” distance between two “events” x1 and x2 is ∆s, with 2 i 2 2 2 2 (∆s) = (∆x) (∆x)i = (c∆t) − (∆x) − (∆y) − (∆z) (5) where ∆x = x2 − x1. 2 I When (∆s) > 0, the two events are said to have a “timelike” separation: they are causally connected, i.e. one can influence the other, and the time ordering of these events is the same in all frames. 2 I When (∆s) < 0, the two events are said to have a “spacelike” separation: they are not causally connected, and one cannot influence the other. The time ordering of these events is frame-depedent. 2 I (∆s) = 0 is the “null cone”, or the light cone. Electromagnetic waves in vacuum travel on this cone. More Lorentz-invariant scalar products Many Lorentz-invariant scalar products of 4-vectors will play an important role in our discussions. m I @ @m = is the D’Alembertian. We have earlier explicitly verified its frame-dependence. m 2 2 2 2 I p pm = (E=c) − j~pj = m c , clearly invariant since it is the mass of the particle. m I pmx = Et − ~p · ~x = ~φ, the phase of a plane wave. We used this quantity while determining the aberration and Doppler shift. m @(ρc) ~ I @mJ = @(ct) + r · J = 0 is the continuity condition / conservation of charge. m @(φ/c) ~ I @mA = @(ct) + r · A = 0 is the Lorentz gauge condition, which was also a frame-independent statement, though we did not bother about it then. Coming up... Metric and invariant scalar products Second rank 4-tensors: symmetric and antisymmetric Higher-rank 4-tensors Definition of a 4-tensor I A second rank 4-tensor B is the object that transforms under frame changes like the direct product of two 4-vectors. mn I The contravariant components B of B should transform as 0mn m n k` B = Λ k Λ ` B (6) and its covariant components Bmn should transform as 0 ¯k ¯` Bmn = Λ mΛ nBk` : (7) m One can also express B in terms of its “mixed” components B n n or Bm , which satisfy appropriate transformation conditions. m I Bm, the trace of B, is a Lorentz invariant. I The Λ’s here are composed of proper rotations and boosts. Space reflections are not included. However we know how elements of direct products of 4-vectors behave under space reflections, since we know how 4-vectors behave under space reflections. The tensors that behave like these are called “proper tensors”, the ones that do not are “pseudotensors” Symmetric second-rank tensors: Λ; Λ¯; g I Till now, we have treated Λ to be simply a matrix. However note m 0m n that Λ n = @x =@x transforms like mixed components of a 4-tensor, and hence Λ is a 4-tensor. 0 I The defining equation for a 4-vector, X = ΛX is then not simply a matrix equation, but a tensor equation. ¯m m 0n I Similarly, Λ n = @x =@x transform like mixed components of a 4-tensor, hence Λ¯ is also a 4-tensor. n mn m I Also, gmn = @xm=@x and g = @x =@xn transform like covariant and contravariant components of a 4-tensor, g. The m m n m mixed components of this tensor areg n = @x =@x = δn . I Sinceg is a tensor, the operation of raising and lowering indices in a 4-vector (or, by extension, a 4-tensor) is a tensor operation. I All second rank tensors can be written as a sum of a symmetric and an antisymmetric tensor. The tensorg is always symmetric, while Λ; Λ¯ are symmetric for only boosts, but are not so when rotations are involved. Now let us look at antisymmetric tensors. Antisymmetric second-rank 4-tensor I An antisymmetric second-rank tensor B may be written in terms of its contravariant components as 0 0 B01 B02 B031 01 12 13 mn B−B 0 B B C B = B C : @−B02 −B12 0 B23A −B03 −B13 −B23 0 There are six independent components, which transform as 0mn m n k` B = Λ k Λ `B : I If we look at the transformation properties of the components B0α (α 2 f1; 2; 3g are the space indices), one obtains 00α 0 α ρµ B = Λ ρΛ µB : 0 Consider space rotations, for which Λ ρ = 1 when ρ = 0 and vanishes otherwise. Then one gets 00α α 0µ B = Λ µB : Thus, the componentsB 0α behave like a 3-vector under rotation. (Note: B is a proper tensor, not a pseudotensor.) Antisymmetric second-rank tensor: general properties αβ I Now let us look at the other components, of the form B . With the advantage of hindsight, consider the quantity 1 αβ Wµ = 2 αβµB where is the completely antisymmetric, Levi-Civita 3-tensor. I The transformation properties of Wµ under space rotations can be obtained as: 1 1 W 0 = 0 B0αβ = Λ¯ν Λ¯ρ Λ¯σ Λα Λβ Bλδ µ 2 αβµ 2 α β µ νρσ λ δ 1 1 = δν δρΛ¯σ Bλδ = Λ¯σ Bλδ 2 λ δ µ νρσ 2 µ λδσ ¯σ = Λ µWσ : Thus, W acts like a vector under proper rotations. Moreover, since B is a proper tensor and is a pseudotensor, W is a pseudovector / axial vector. I Thus, the six independent components of the antisymmetric second-rank tensor can be separated into α 0α a 3-vector V = B = (Vx ; Vy ; Vz ), and αβ a 3-axial vector Wµ = αβµB = (Wx ; Wy ; Wz ). Vector ⊕ axial vector ) antisymmetric tensor I In terms of the vector V and the axial vector W , one can write the components of Bij as 0 1 0 Vx Vy Vz mn B−Vx 0 Wz −Wy C B = B C : @−Vy −Wz 0 Wx A −Vz Wy −Wx 0 I This should remind us of the electromagnetic field tensor F, whose components are written in terms of E~ , a vector, and B~ , an axial vector: 0 1 0 −Ex −Ey −Ez mn BEx 0 −Bz By C F = B C : (8) @Ey Bz 0 −Bx A Ez −By Bx 0 We shall later devote a complete lecture to the exploration of F. Coming up... Metric and invariant scalar products Second rank 4-tensors: symmetric and antisymmetric Higher-rank 4-tensors Rank-n tensors and completely antisymmetric th I Along the same lines as before, an n rank tensor is an object whose components transform as the direct product of n 4-vectors. The tensors may be represented in terms of their contravariant, convariant, or mixed components. I The 4-d analogue of the Levi-Civita tensor is , whose components are defined through 0123 = +1, and k`mn = ±1 depending on whether (k; `; m; n) is an even or odd permutation of (0; 1; 2; 3). In all the other cases (i.e. when any two or more of k; `; m; n are equal), k`mn = 0. I Components of do not change with proper Lorentz transformations. Moreover, they do not change sign even under space reflections, which is what a proper tensor would be expected to do. Therefore, is a pseudotensor. I Quantities that are made up from a product of tensors and a single are pseudotensors. We shall come across a few of them later in this course. Dual tensors with Because of its completely antisymmetric nature, plays an important role in creating “dual” tensors. For example, k`m 1 k`mn I If X is a vector, Xe defined through Xe = 2 Xn is a rank-3 pseudotensor, which is completely antisymmetric in its three indices. I If X is an antisymmetric rank-2 tensor, Xe defined through k` 1 k`mn Xe = 2 Xmn is a rank-2 antisymmetric pesudotensor.
Details
-
File Typepdf
-
Upload Time-
-
Content LanguagesEnglish
-
Upload UserAnonymous/Not logged-in
-
File Pages17 Page
-
File Size-