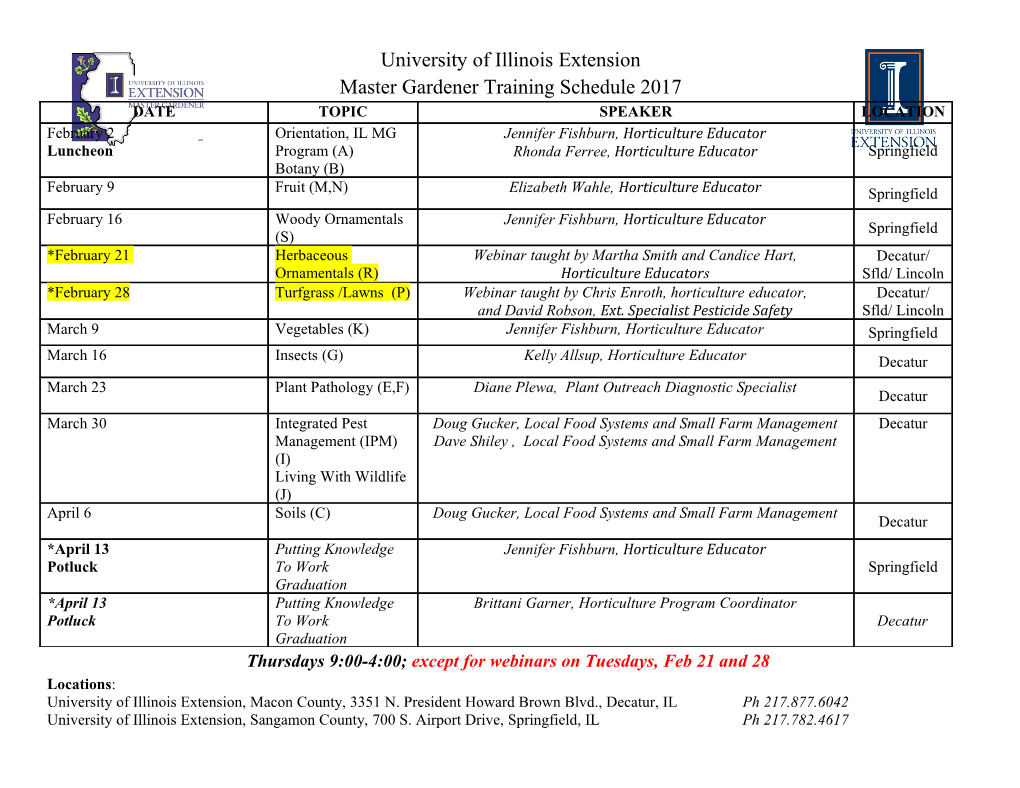
MANTLE CONVECTION IN TERRESTRIAL PLANETS Elvira Mulyukova & David Bercovici Department of Geology & Geophysics Yale University New Haven, Connecticut 06520-8109, USA E-mail: [email protected] May 15, 2019 Summary surfaces do not participate in convective circu- lation, they deform in response to the underly- All the rocky planets in our Solar System, in- ing mantle currents, forming geological features cluding the Earth, initially formed much hot- such as coronae, volcanic lava flows and wrin- ter than their surroundings and have since been kle ridges. Moreover, the exchange of mate- cooling to space for billions of years. The result- rial between the interior and surface, for exam- ing heat released from planetary interiors pow- ple through melting and volcanism, is a conse- ers convective flow in the mantle. The man- quence of mantle circulation, and continuously tle is often the most voluminous and/or stiffest modifies the composition of the mantle and the part of a planet, and therefore acts as the bottle- overlying crust. Mantle convection governs the neck for heat transport, thus dictating the rate at geological activity and the thermal and chemical which a planet cools. Mantle flow drives geo- evolution of terrestrial planets, and understand- logical activity that modifies planetary surfaces ing the physical processes of convection helps through processes such as volcanism, orogene- us reconstruct histories of planets over billions sis, and rifting. On Earth, the major convective of years after their formation. currents in the mantle are identified as hot up- wellings like mantle plumes, cold sinking slabs and the motion of tectonic plates at the surface. On other terrestrial planets in our Solar System, Keywords mantle flow is mostly concealed beneath a rocky surface that remains stagnant for relatively long Mantle convection, terrestrial planets, planetary periods of time. Even though such planetary evolution, plate tectonics, volcanism 1 Oxford Research Encyclopedia of Planetary Science Mantle Convection Mantle Convection In planetary mantles, the convective currents can deform and chemically modify the top and The interiors of terrestrial planets are comprised bottom boundaries; their effect on the planetary of three main layers: a metallic core at the surfaces is of particular interest, since that center, overlain by a rocky mantle, which part can be most readily observed and used is in turn enveloped by a rocky crust. The to interpret the workings of the underlying exact compositions and thicknesses of these mantle. For example, hot upwelling mantle layers, and their thermal and chemical evolu- currents can generate surface uplift, seen as tion through time, vary from planet to planet, topographic highs, or induce volcanic activity, depending on their size, distance from the sun, when hot material melts and erupts while formation history, etc. However, common to all approaching the surface. Extruded lavas on the terrestrial planets in our Solar System, and planetary surfaces record the presence and even to some of its larger moons, is that their evolution of hot mantle regions, and can be mantles undergo convective motions, wherein used to infer mantle temperature, chemistry hot buoyant material rises from the deep interior and flow velocity. Similarly, the downwelling and the heavy cold material near the surface currents can give rise to topographic lows, as sinks. the sinking mantle material pulls the surface down from below. In the possibly unique case Mantle convection is the dominant mech- of the Earth, the top cold thermal boundary anism by which planets cool and undergo layer is subdivided into tectonic plates, which chemical segregation. The flow of the mantle are moving relative to each other and sink into induces motion in the overlying crust, which the mantle at subduction zones. The rate at can lead to such phenomena as volcanoes, which a planet recycles cold material into the earthquakes and, uniquely for Earth, plate tec- mantle largely determines its cooling rate. tonics. Ultimately, mantle convection governs the evolution of planetary surfaces and interiors. Naturally, more observations are available for the Earth’s surface and mantle than for other The fundamental features of any convective planets. Thus, our understanding of planetary system include cold and hot boundaries (such interiors, and their surface manifestations, is as the outer and inner boundaries of the mantle, largely shaped by what is known about our respectively), and a fluid between the two home planet, as well as by our understanding of boundaries on which gravity acts to move hot the fundamental processes that govern mantle and cold material. Hot upwelling and cold convection, such as the physics of heat transport downwelling vertical currents are connected and rock deformation. along the horizontal boundaries by the hot and cold thermal boundary layers (TBLs): a In what follows, the different components of hot TBL at the bottom, and a cold TBL at the convective mantle flow on Earth are described, top. The TBLs are where heat is conducted tracking the material trajectory as it forms tec- rapidly across the boundaries into or out of the tonic plates traversing the Earth’s surface, which convectively stirred mantle. The large thermal then sink into the mantle as cold subducting gradients across the TBLs, compared to if the slabs, that eventually impinge on and flow lat- temperature increased gradually from top to erally along the core-mantle boundary; some of bottom, is what makes thermal convection such this material ascends across the mantle again as an efficient mechanism for heat transfer. mantle plumes, while most of it ascends broadly as part of the global tectonic circulation, thus 2 Oxford Research Encyclopedia of Planetary Science Mantle Convection closing the loop. The convective currents on they get partially reflected, and these reflected other terrestrial planets are discussed as well, signals allow to determine the boundaries of the albeit our understanding of these is less certain main layers that make up the Earth’s interior. due to fewer observational constraints. We will Furthermore, hot rock is typically softer and then survey the underlying physics of convec- more easily compressed, hence seismic waves tion, which forms the basis for understanding are slower passing through such material; how mantle convection is both similar to and the opposite is true for cold rocks which are different from classical theories of convective stiffer. The resulting seismic wave travel time flow, and how this physics lets us infer mantle variations can be used to infer pictures of the dynamics on Earth and other terrestrial planets. mantle showing “hot” (seismically slow) and “cold” (fast) regions, appearing much like an ultrasound of the mantle. Mantle Top to Bottom The distance to the center of the Earth is One of the greatest challenges in the studies approximately 6400 km, of which the mantle of planetary mantles is their inaccessibility comprises about 2900 km, sandwiched between for direct observations. The structure and the thin crust (average thickness of about 20 physical properties of planetary interiors have km: 7 km in the ocean and 40 km in continents) to be inferred from indirect measurements such and iron core (about 3500 km radius). Although as satellite observations of gravity, surface the core is thicker, the mantle envelops it and topography and magnetic fields [Phillips and thus the mantle constitutes about 80% of our Ivins, 1979], see Sohl and Schubert [2015] planet’s volume (Figure 3). The similar size for a recent review. In addition, analysis of and density of Earth and Venus, which has meteorites collected at the Earth’s surface a total radius of about 6100 km, makes it constrain the chemistry of some other planets, likely that the thicknesses of the venusian core as well as the building blocks of our own and mantle are similar to those of Earth. At planet. Earth is special in this sense, because, in abour 3400 km total radius, Mars is the third addition to the remote measurements, scientists largest terrestrial body in the Solar system. The have access to a wealth of geological samples combined measurements of the martian mass, and can perform seismological observations moment of inertia (i.e., inertial resistance to of the interior. Most of what we know about being spun), and chemical analysis of martian Earth’s interior is obtained indirectly from the meteorites, constrain the radius of the martian analysis of seismic waves, which are triggered core to be about 1400 km, leaving about 1900 by powerful earthquakes and propagate through km to be taken up by the mantle, and 100 km the mantle and core. Seismic waves travel faster by the crust [Harder, 1998]. The radial mass through rocks that are stiffer. In the mantle, the distribution of Mercury is unusual, compared rocks become denser, and therefore stiffer, when to other terrestrial bodies, in that most of it is they are exposed to higher pressures at greater occupied by a dense metallic core, which is depths. The resulting increase with depth of the about 2020 km in in radius, overlain by a 400 measured seismic velocities can thus be used to km thick mantle [Hauck et al., 2013] and a 50 infer mantle’s density structure. When seismic km thick crust [Smith et al., 2012]. waves pass through sharp changes in material properties (e.g., density), such as the boundaries While the metallic cores likely separated between the felsic crust and the mafic mantle, out of the mantles early in planetary histories, or the silicate mantle and the metallic core, i.e., within the first few tens of Myr of the life 3 Oxford Research Encyclopedia of Planetary Science Mantle Convection Venus Earth Mars Mercury Figure 1: Cutaway views of the interiors of four terrestrial planets in the Solar System. Reproduced from http://solarviews.com/cap/index/cutawaymodels1.html of the Solar System [Kleine et al., 2002], the crust.
Details
-
File Typepdf
-
Upload Time-
-
Content LanguagesEnglish
-
Upload UserAnonymous/Not logged-in
-
File Pages52 Page
-
File Size-