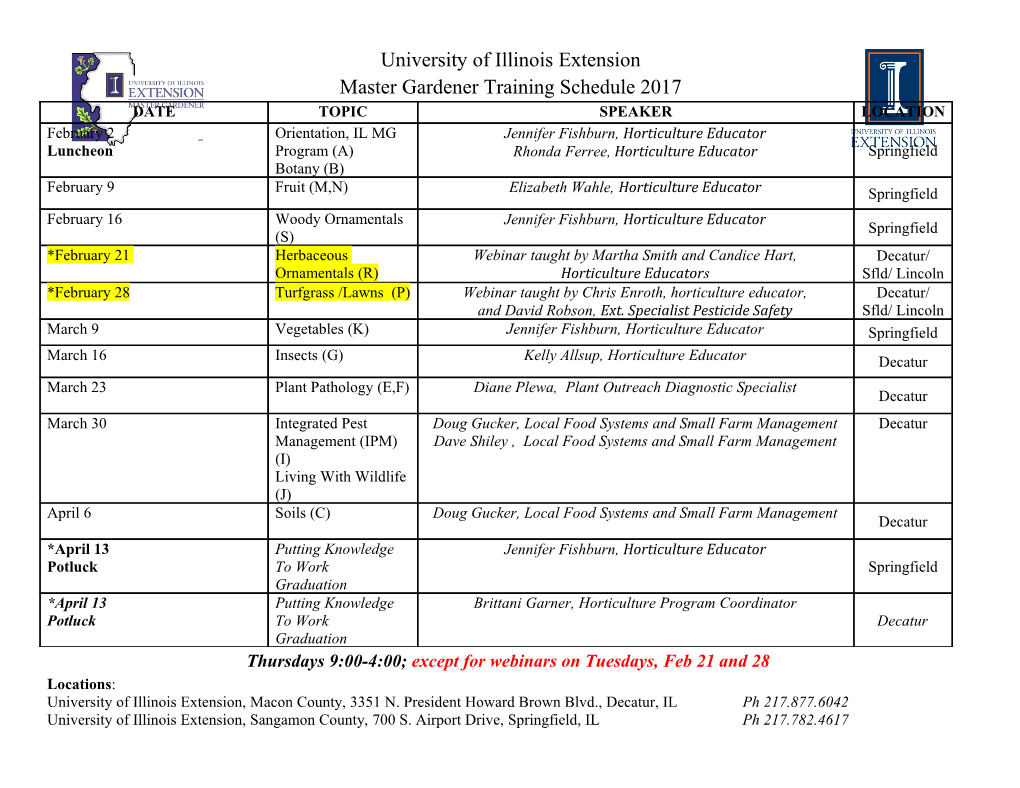
Perspective Relating Catalysis between Fuel Cell and Metal-Air Batteries Matthew Li,1,2 Xuanxuan Bi,1 Rongyue Wang,3 Yingbo Li,4,6 Gaopeng Jiang,2 Liang Li,5 Cheng Zhong,6,* Zhongwei Chen,2,* and Jun Lu1,* With the ever-increasing demand for higher-performing energy-storage sys- Progress and Potential tems, electrocatalysis has become a major topic of interest in an attempt to Catalyst research for fuel cells has enhance the electrochemical performance of many electrochemical technolo- led to much advancement in gies. Discoveries pertaining to the oxygen reduction reaction catalyst helped humanity’s understanding of the enable the commercialization of fuel-cell-based electric vehicles. However, a underlying physics of the process, closely related technology, the metal-air battery, has yet to find commercial significantly enhancing the application. Much like the Li-ion battery, metal-air batteries can potentially uti- performance of the technologies. lize the electrical grid network for charging, bypassing the need for establishing In contrast, metal-air batteries a hydrogen infrastructure. Among the metal-air batteries, Li-air and Zn-air bat- such as Li-air and Zn-air batteries teries have drawn much interest in the past decade. Unfortunately, state-of-the remain to be solved. Although the art metal-air batteries still produce performances that are well below practical metal anode used in this these levels. In this brief perspective, we hope to bridge some of the ideas from systems does play a large role in fuel cell to that of metal-air batteries with the aim of inspiring new ideas and di- limiting their commercial success, rections for future research. catalysis also remains quite challenging. In this perspective, a INTRODUCTION discussion is provided on the similarities and differences Increasing the energy density of the battery system on board electric vehicles (EVs) between metal-air catalysts and has become a major topic of research interest throughout the electrocatalysis com- fuel cells for aqueous (alkaline/ munity.1–3 The development of catalysts that efficiently facilitate the oxygen reduc- acidic) and aprotic electrolytes. By tion reaction (ORR) allowed for great commercial advances in the field. Recent appli- attempting to bridge the cation of fuel cell technologies in EVs has led to the commercialization of a variety of discussion between the fields and fuel cell vehicles such as the Toyota Mirai.4 This EV boasts an attractive cruising providing our own opinion on the range of 500 km, cold start capability, and a significantly shorter refueling time subject, we hope that this of 3 min when compared with battery-based EVs.5 Unfortunately, the price remains perspective will present itself as a relatively high (7 million Japanese yen or 57,500 USD in 20144) due to the large starting point in emulating the impact of the Pt-based catalyst on the cost of the vehicle and the low production vol- success of catalysis in fuel cells in umes.6,7 While this might be within the purchasing power of some consumers (as a the metal-air systems. luxury vehicle), it will unlikely become widespread at this price point. Beyond the development of vehicle cost, a country- or even a city-wide hydrogen infrastructure is still far from realization and is considered a major hurdle against the widespread adoption of hydrogen fuel cells in the transportation sector. In contrast to fuel cell technologies, the closely related metal-air batteries (MABs) can be seen as a decent compromise between lithium-ion batteries (LIBs) and hydrogen fuel cell systems. Theoretically, MABs such as the Li-air battery (LAB) should have drastically higher energy densities when compared with a LIB. While cal- culations taking into account the volume of some non-active material have led to more modest volumetric energy density estimates (less than LiCoO2-based LIBs), the gravimetric energy density has beencalculatedtobetwicethatofLIBs.8 More- over, the electricity infrastructure needed to recharge MABs will likely be able 32 Matter 2, 32–49, January 8, 2020 ª 2019 Elsevier Inc. to bootstrap the already present and quickly growing EV charging infrastructure (be it charging stations or potential future battery-swapping stations9). Although this is perhaps one of the most important advantages of MABs, it will inevitably come at the cost of energy density when compared with the hydrogen fuel cell. It is, however, important to note that this range disadvantage has already been rather well toler- ated by current LIB-based EV consumers, indicating that the energy difference be- tween MABs and hydrogen fuel cells might not be a polarizing deciding factor. The difference in recharge/refuel time between MABs and fuel cells will likely decrease with more standardization among battery-pack designs, thus promoting battery-swapping stations.9 With many candidate MAB systems for EVs such as LABs and Zn-air batteries (ZABs) among other MAB systems still suffering from unre- solved technical challenges,10–14 the future applications of MABs remain unclear. Although the commercial application of fuel cells in EVs has already gained substan- tial progress in recent years, most MABs have remained in their conceptual/proto- type stages. The clear difference in maturity between the two systems suggests very different technical challenges. On the surface, the MABs appear to be quite similar to fuel cells and are sometimes referred to as semi-fuel cells.15,16 Like the hydrogen fuel cells, all MABs also require an external oxidizing agent (O2)thatis ideally accessed from the ambient air, separate from the inherent mass and volume of the MAB system. Although there are many technical challenges associated with using a metal anode,17,18 the seemingly similar cathode between these systems have subtle yet significant differences. Variations in the nature of the discharge reac- tion and product, such as the solid/poorly soluble and insulating Li2O2 formed dur- ing the discharge of LABs19 versus the liquid water produced in hydrogen fuel cells, have led to a great divergence in their research strategies. While some researchers have attempted to relate the different systems, it is difficult to foresee how they will intersect.20–22 If clear analogies can be drawn between these systems, new para- digms in research can be devised. Therefore, it is timely to compare and contrast the different meaning of catalyst between these technologies, proposing a situation whereby similar design concepts can be used. We will first elaborate on the differ- ences and similarities between ZAB, LAB, and fuel cell catalysis in aqueous media. 1Chemical Sciences and Engineering Division, Argonne National Laboratory, 9700 Cass Avenue, We will then compare these technologies in aprotic media and propose operational Lemont, IL 60439, USA conditions whereby more similarities can be found and leveraged. 2Department of Chemical Engineering, Waterloo Institute of Nanotechnology, University of Waterloo, 200 University Avenue West, Waterloo, AQUEOUS SYSTEMS ON N2L 3G1, Canada 3 Acidic Media Applied Materials Division, Argonne National Laboratory, 9700 Cass Avenue, Lemont, IL 60439, Among all the contemporary technologies for electrocatalysis, the most commer- USA cially successful is the application in the acidic medium hydrogen fuel cell, more 4Materials Science Division, Argonne National commonly known as the proton exchange membrane (PEM) fuel cell. Although Laboratory, 9700 Cass Avenue, Lemont, IL 60439, USA acid medium is not preferable for MABs (due to corrosion of Zn), it is important to 5College of Physics, Optoelectronics and Energy, contrast the catalytic mechanism. In acidic electrolyte fuel cells, it is commonly Center for Energy Conversion Materials & Physics accepted that an adsorbed oxygen will reduce to an adsorbed OH* or OOH* before (CECMP), Soochow University, Suzhou 215006, P. 23 R. China further reduction to H2O. During this process, the breakage of the O–O bond is 6 typically the rate-limiting step that requires a catalyst. Only by lowering the overpo- Key Laboratory of Advanced Ceramics and Machining Technology (Ministry of Education) tential associated with ORR can PEM fuel cells operate at sufficient energy effi- and Tianjin Key Laboratory of Composite and Functional Materials, School of Materials Science ciencies and power densities. The ORR begins with the adsorption of O2 molecules and Engineering, Tianjin University, Tianjin onto the catalytically active sites on the surface of platinum. The next step is the 300072, China weakening of the oxygen bond and reduction of the molecule by protons and elec- *Correspondence: trons forming an adsorbed OOH* followed by a series of steps and intermediates [email protected] (C.Z.), (O*, OH*), finally forming water.23 The overall process is presented in Figure 1A. [email protected] (Z.C.), [email protected] (J.L.) The reaction kinetics is largely controlled by the degree to which the intermediates https://doi.org/10.1016/j.matt.2019.10.007 Matter 2, 32–49, January 8, 2020 33 Figure 1. Properties of Oxygen Reduction Reaction (A) O2 is shown to reduce to H2O with the various reaction rate constants denoted with a numbered k. Reproduced with permission from MarkovicandRoss.23 Copyright 2002, Elsevier. (B) Volcano plot for ORR activity versus HO* adsorption energy for various catalysts. On the left axis, Pt a plot of activity enhancement relative to Pt at U = 0.9 V (RHE) versus DGHO* À DG HO*,andonthe right axis a plot of U required for all reaction steps to be negative in free energy versus DGHO* À Pt 24 DG HO* Reproduced with permission from Stephens et al. Copyright 2012, Royal Society of Chemistry. are adsorbed by the catalyst surface. One would want a catalyst surface with the optimal adsorption energy for every intermediate to achieve the best catalytic activ- ity. However, the binding energies of all the intermediates in ORR are governed by what is known as a linear scaling relation (LSR).24 LSR refers to the phenomenon whereby moving toward a stronger adsorption of OOH* (one intermediate) to facil- itate the dissociation of O2 molecule will actually result in a less favored subsequent reduction and desorption of OH* (another intermediate).
Details
-
File Typepdf
-
Upload Time-
-
Content LanguagesEnglish
-
Upload UserAnonymous/Not logged-in
-
File Pages18 Page
-
File Size-