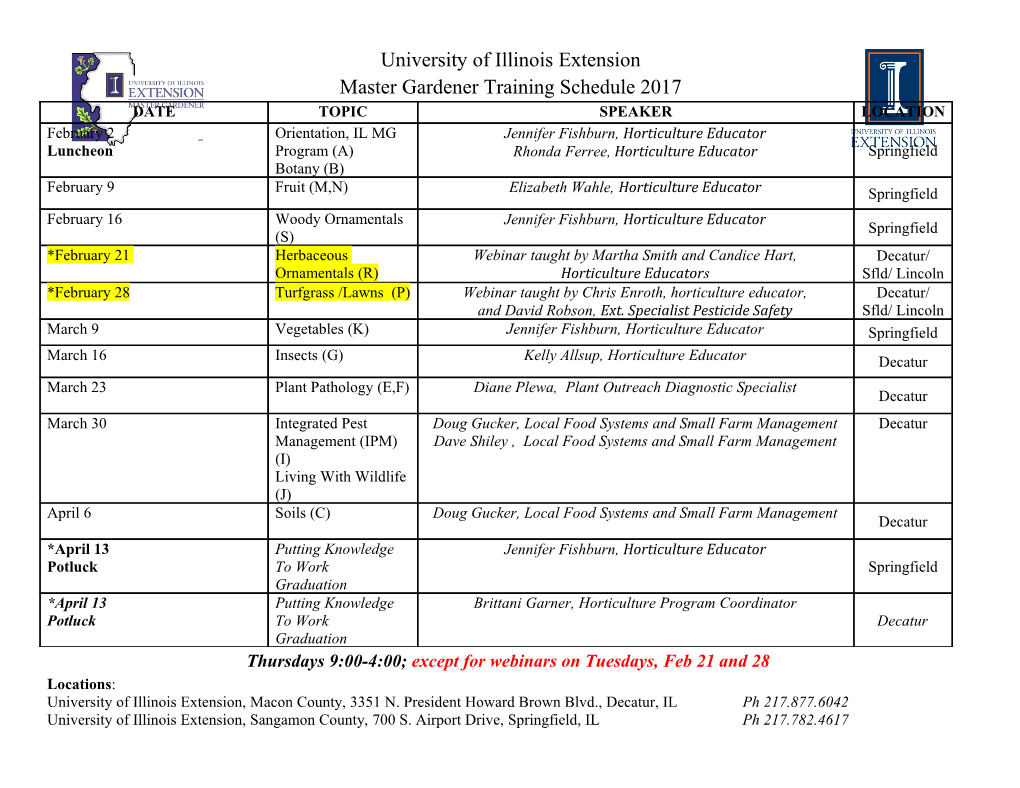
Seventh International Conference on Mars 3156.pdf MARS LATITUDE, MARS OBLIQUITY, AND HYDRATION STATES OF Mg-SULFATES. D. T. Vani- man1, S. J. Chipera1, D. L Bish2, and R. C. Peterson3, 1Group EES-6, MS D462, Los Alamos National Laboratory, Los Alamos, NM 87545 ([email protected]; [email protected]), 2Dept. of Geological Sciences, Indiana Univ., 1001 E. 10th St., Bloomington, IN 47405 ([email protected]), 3Dept. of Geological Sciences and Geological Engi- neering, Queen’s Univ., Kingston, Ontario, K7L 3N6, Canada ([email protected]). Introduction: Mg-sulfate minerals and synthetic phases exist in many hydration states, from anhydrous forms that are synthesized commercially to the com- mon retail product epsomite (MgSO4·7H2O) and the newly described synthetic 11-hydrate [1] and its natu- ral equivalent [see abstract by Peterson in this vol- ume]. Table 1 summarizes a variety of Mg-sulfate forms as described in [1] and [2]. Table 1: Forms of Mg-sulfate hydrates Formula Mineral name MgSO4 anhydrous none (reagent) MgSO4·1H2O kieserite MgSO4·1H2O type 2 none (reagent) MgSO4·2H2O sanderite MgSO4·2.4H2O none MgSO ·4H O starkeyite 4 2 MgSO4·5H2O pentahydrite The 11-hydrate form of Mg-sulfate (Figure 1) may MgSO4·6H2O hexahydrite be a stable phase on Mars under current higher-latitude MgSO4·7H2O epsomite conditions where water ice is present to maintain high MgSO4·11H2O pending approval by IMA* relative humidity, albeit at very low pH2O. At lower MgSO4·nH2O amorphous (n variable) latitudes, where daytime relative humidity is very low, *International Mineralogical Association the more likely form of Mg-sulfate hydrate may be either kieserite or an amorphous form with water con- It is conceivable that any of these Mg-sulfate tent dependent at least in part on temperature [5,6]. forms may occur on Mars. Although several can only Between these two extremes is a wide, cold region form metastably [2] and some require relatively high between essentially 0% and 100% relative humidity temperatures for formation, conditions of burial, im- where phase transitions are poorly known, but both pact, volcanic heating, and surface exposure provide a desiccation and hydration reactions may lead to a vari- great variety of temperature-pH2O conditions on Mars. ety of hydrated forms. In this paper we are most concerned with surface con- Desiccation: The non-equilibrium desiccation of ditions that are typically cold with variable vapor pres- several grain sizes of epsomite at two different tem- sure of water. Figure 1 shows the reaction boundary peratures and a pH2O of ~0.7 Pa is summarized in Fig- between epsomite and hexahydrite [3], the reaction ure 2 (from reference [5]). The water-loss data are boundaries between hexahydrite and starkeyite or shown as both wt% and as equivalent stages of water starkeyite and the monohydrates (kieserite and reagent loss from n=7 to n=0, where n represents the number monohydrate) [2], and the reaction boundary between of water molecules in the formula MgSO4·nH2O. XRD epsomite and the 11-hydrate [1]. Figure 1 is also con- analysis shows that at these conditions the epsomite toured for pH2O in Pascals (Pa). Included in Figure 1 starting material becomes X-ray amorphous between is a shaded region showing day-night temperature and 24 and 33 hrs. The weight-loss curves show little dif- relative humidity variation at the Viking 1 site in late ference between the five size fractions after ~2-10 summer [4] and a field indicating the high-latitude hours of desiccation. At both temperatures a point is conditions where near-surface water ice may persists ultimately reached where prolonged exposure to this on Mars today (blue field along the right edge). low-pH2O condition results in little or no additional dehydration, although there is a significant temperature Seventh International Conference on Mars 3156.pdf effect with higher residual water content at lower tem- point slightly below n=7, followed by slower hydration perature. through and beyond n=7 at ~0.048 wt.%/day. Also shown in Figure 3 are initial data from a sec- Figure2:Desiccation of epsomiteat two temperatures with ond experiment with the n=1.2 amorphous precursor pH O~0.7Pa. 2 over water ice at 220 K. As expected for a much lower 0 297 K temperature with lower pH2O, the initial hydration rate 323 K n=6 10 is slow (0.04 wt.%/day) but comparable to the second- n=5 stage hydration rate for a kieserite precursor beyond ) 20 % n=4 n=7 at 243 K. At 220 K the rehydration of the amor- ( 4 s s phous form is expected to take ~4.8 x 10 hours (~3 o n=3 l 30 t Mars years) to reach n=7; whether there is pause with h g i n=2 e “soak time” at this point followed by continued hydra- w 40 <45 μm n=1 tion toward the 11-hydrate is not known. If the initial 45-75 μm 50 75-180 μm rate at 220 K is maintained, hydration of the amor- 180-425 μm n=0 425-850 μm phous form to n=11 will take ~5-6 Mars years. 2mm 60 0.1 1 10 100 1000 Samples of hydrating kieserite at 243 K were ana- time (hours) lyzed by X-ray diffraction to test whether the 11- hydrate structure determined by [1] accounts for in- Figure 3: Hydration of amorphous (n = 1.2) and kieserite (n = 1) samples over water ice at 243 K or 220 K. Solid horizontal lines indicate hydration to creased hydration beyond n=7. As the 11-hydrate n = 7 or 11 (delta from amorphous precursor); dashed horizontal lines structure is unstable at temperatures >275 K, the sam- indicate equivalent hydration for kieserite precursor. ples were analyzed on a metal sample mount chilled by 140 a liquid nitrogen reservoir (Figure 4a,b). The diffrac- nδkies =11 tion pattern obtained (Figure 5) shows that both ep- n =11 120 δamorph somite and the 11-hydrate are present, confirming hy- dration of kieserite first to epsomite and then to the 11- K 43 at 2 hydrate. 100 sor cur K re t243 s p sor a hou recur orp rite p n e i am kies Figure 4a a 80 g nδkies =7 % nδamorph =7 . t W 60 40 20 t 220 K cursor a ous pre amorph 0 0 5000 10,000 15,000 20,000 time (hours) Hydration: Hydration of an amorphous form of Mg-sulfate with n=1.2, as produced at 297 K (Figure 2), is depicted in Figure 3, which shows the hydration reaction under pH2O conditions controlled by water vapor in equilibrium with water ice at 243 K and 220 K (yellow triangles). Figure 3 also shows a hydration curve for kieserite in vapor communication with water ice at 243 K (red squares). The data at 243 K show that the amorphous material, even though coarser-grained than the kieserite (75-180 μm vs. <45 μm), rehydrates more rapidly. At 243 K the amorphous precursor also shows a “soak time” of ~30 days at epsomite composi- tion (n=7) before weight gain resumes beyond n=7. At 243 K the kieserite precursor does not have a compa- rable “soak time” at n=7 but shows two hydration re- gimes, an initial rapid hydration (~0.38 wt.%/day) to a Seventh International Conference on Mars 3156.pdf Figure 4b indicate that at all temperatures and at all hydration states the hydration rates for kieserite are slower (by a factor of ~1.2 to 1.3) than for the amorphous form. The increased hydration rate of amorphous MgSO4·nH2O suggests that it is in an “activated” state relative to kieserite. Figure 6: Hydration times at various temperatures, starting with either kieserite (n = 1) or an amorphous Mg-sulfate (n =1.2)andpH2O controlled by water ice. 105 yrs ??ice blanket above 105 yrs +60ο latitude [9] 104 yrs 104 yrs black curves = amorphous precursor red curves = kieserite precursor 103 yrs 103 yrs 2 10 yrs e e 102 yrs m m t i i im t t t ime e h s to t r 10 yrs tor re r a a 10 yrs e a a ch M c E h n n = 1yr = 1 7 1 1yr 69 days seasonal 37 days frost at 6.9 days mid-latitudes [7,8] 3.7 days 17 hrs 180 230 280 330 temperature (K) On Figure 6 we have plotted durations and average temperatures for mid-latitude frost accumulation on Mars at present obliquity [7,8] and at latitudes pole- From data reported in [5] and the data summarized ward of 60° by redistribution of ice after high- in Figure 3 it is possible to construct curves of hydra- obliquity accumulation at Tharsis [9]. Extrapolated tion rates from the amorphous form and from kieserite curves for n=7 and n=11 suggest that seasonal frost in vapor communication with water ice at various tem- accumulation over a winter at mid-latitudes does not peratures (Figure 6). Data points for hydration to the allow sufficient time to hydrate either the amorphous 7-hydrate (epsomite) from an amorphous precursor form or kieserite to n=7 or n=11, but a blanket of ice with n=1.2 are shown as open circles; those for hydra- above 60° latitude following an episode of higher tion to epsomite from kieserite are shown as red trian- obliquity may be of sufficient duration for hydration to gles. n=11. However, this conclusion assumes that extrapo- Extrapolated times for hydration to the 11-hydrate lation of available data to below 220 K is valid.
Details
-
File Typepdf
-
Upload Time-
-
Content LanguagesEnglish
-
Upload UserAnonymous/Not logged-in
-
File Pages4 Page
-
File Size-