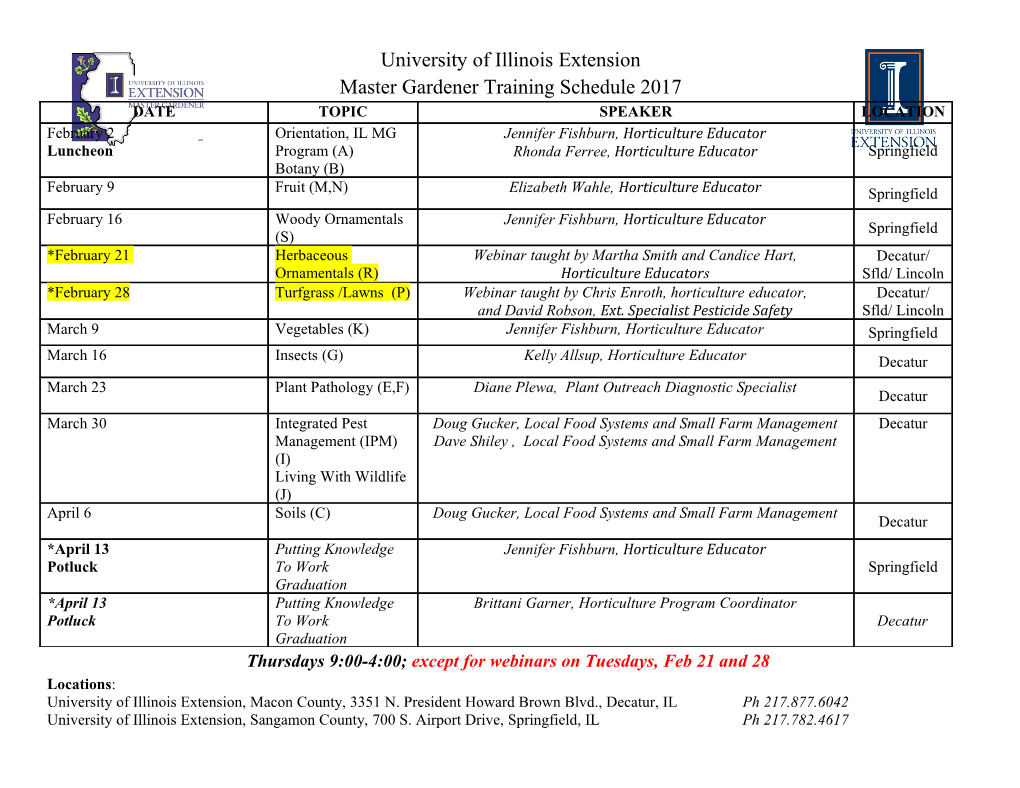
Downloaded from orbit.dtu.dk on: Oct 04, 2021 Homogeneous Charge Compression Ignition Combustion of Dimethyl Ether Pedersen, Troels Dyhr Publication date: 2011 Document Version Publisher's PDF, also known as Version of record Link back to DTU Orbit Citation (APA): Pedersen, T. D. (2011). Homogeneous Charge Compression Ignition Combustion of Dimethyl Ether. Technical University of Denmark. General rights Copyright and moral rights for the publications made accessible in the public portal are retained by the authors and/or other copyright owners and it is a condition of accessing publications that users recognise and abide by the legal requirements associated with these rights. Users may download and print one copy of any publication from the public portal for the purpose of private study or research. You may not further distribute the material or use it for any profit-making activity or commercial gain You may freely distribute the URL identifying the publication in the public portal If you believe that this document breaches copyright please contact us providing details, and we will remove access to the work immediately and investigate your claim. - 1 - - Homogeneous Charge Compression Ignition Combustion of Dimethyl Ether PhD Thesis Troels Dyhr Pedersen DTU Mechanical Engineering Department of Mechanical Engineering - 2 - - Table of contents 1 FOREWORD 6 2 NOMENCLATURE 7 3 RÉSUMÉ 8 4 RESUMÉ (DANSK) 10 5 INTRODUCTION 12 5.1 About HCCI combustion 12 5.2 Background of this project 13 5.3 Scope of investigation 14 5.3.1 The study on combustion phasing 14 5.3.2 The study on combustion noise 15 6 THE BASICS OF HCCI COMBUSTION 16 6.1 Combustion principle 16 6.2 Combustion characteristics 16 6.3 Combustion phasing parameters 17 6.3.1 Compression ratio 17 6.3.2 Equivalence ratio 17 6.3.3 Inlet temperature 17 6.3.4 Exhaust gas recirculation 17 6.4 Thermal efficiency 18 6.5 Emission characteristics 18 7 COMBUSTION OF DME 20 7.1 Combustion stoichiometry 20 7.2 Physical and chemical properties of DME 20 7.3 Development in the use of dimethyl ether as a fuel 21 8 DESCRIPTION OF EXPERIMENTAL ENGINE SETUP 22 8.1 Engine 22 - 3 - - 8.1.1 Engine setup 22 8.1.2 Modifications 22 8.1.3 Piston modifications 24 8.2 Cylinder pressure transducers 25 8.3 Data acquisition 25 9 HEAT RELEASE CALCULATIONS 27 9.1 The Heat Release analysis 27 9.2 Location of TDC 27 9.3 Specific heat ratio 29 9.4 Temperature estimate 31 9.5 Heat losses 31 10 THE REACTION KINETICS OF DME 33 10.1 Elementary reaction model 33 10.2 The detailed reaction scheme 33 10.3 Reducing the mechanism for lean combustion 33 10.4 Comparison of the full and the reduced schemes 36 10.5 Reaction paths 37 10.5.1 Initiation and low temperature reaction paths 37 10.5.2 High temperature reactions 41 10.6 Manipulating low temperature reactions 42 10.6.1 Effect of methanol on low temperature reactions 42 10.6.2 Effect of EGR 43 10.7 List of species 44 11 ENGINE ACOUSTICS 45 11.1 Measurements 45 11.2 Choice of sampling frequency 45 11.3 Equipment 46 11.4 Handling the confined space acoustic issues 46 11.5 Post sample digital filtering 47 11.5.1 Filter methodology 47 11.5.2 Filtering of pressure data for heat release analysis 47 - 4 - - 11.5.3 Filtering of sample data for frequency analysis 48 11.6 Representation of acoustic measurements 48 11.6.1 Sound pressure 48 11.6.2 Sound pressure level 48 11.6.3 Time domain representation 49 11.6.4 Frequency domain representation 50 11.6.5 Frequency spectrum analysis in the time domain 51 11.7 Theory of cylinder acoustics 53 11.7.1 Circumferential modes 53 11.7.2 Radial modes 53 11.7.3 Axial modes 54 11.8 Calculation of resonance frequencies 54 11.9 COMSOL calculations of cylinder acoustic resonance 55 12 SIMULATION OF DETONATION PHENOMENA 58 12.1 Background 58 12.2 Explosions 59 12.2.1 Uniform explosion 59 12.2.2 Non uniform explosions and pressure wave amplification 59 12.3 Detonations 60 12.3.1 Introduction 60 12.3.2 Detonation waves 61 12.3.3 Shock wave 61 12.3.4 Reference for velocitities 61 12.3.5 Conservation equations 63 12.3.6 The Rayleigh relation 63 12.3.7 The Hugoniot relation 64 12.3.8 Determination of the Chapman Jouget velocity 67 12.3.9 Results for the stationary solution 70 12.4 Detonation study in CFD 71 12.4.1 Problem setup in STAR-CD 71 12.4.2 Simulation results 73 12.5 Observations of detonation in HCCI combustion 76 12.5.1 Experimental setup 76 12.5.2 Pressure trace 77 13 SUMMARY AND CONCLUSION 79 14 REFERENCES 81 15 APPENDIX A: REDUCED SCHEME FOR LEAN PREMIXED DME COMBUSTION 84 - 5 - - 16 APPENDIX B: DETONATION MODEL IN EES 86 17 PAPER I : THE EFFECT OF COMPRESSION RATIO, EQUIVALENCE RATIO AND ENGINE SPEED ON HCCI COMBUSTION OF DIMETHYL ETHER 86 18 PAPER II: CONTROLLING THE HEAT RELEASE OF DME HCCI COMBUSTION WITH METHANOL AND EGR 89 19 PAPER III: REDUCING HCCI COMBUSTION NOISE THROUGH PISTON DESIGN 90 - 6 - - 1 Foreword The work described in this thesis was initiated in June 2006 and completed in January 2010. It was carried out under supervision of associate professor Jesper Schramm at the Department of Mechanical Engineering, Technical University of Denmark. I wish to thank Jesper Schramm for offering me the PhD position. I also wish to thank the rest of the Internal Combustion Engine Group. Everyone has been supportive and helpful in many situations. In 2008 I visited the National Traffic Safety and Environmental Laboratories in Tokyo, Japan, for 5 months. The purpose of the visit was to perform experiments with HCCI combustion on a truck engine test bench. I am grateful for having been given this opportunity to work in a professional environment, and wish to thank the people whom I worked with at NTSEL for their help and kindness. - 7 - - 2 Nomenclature AC Alternating Current ASRT Automatic Scheme Reduction Tool ATAC Active Thermo Atmosphere Combustion ATDC After Top Dead Center BMEP Brake Mean Effective Pressure BTDC Before Top Dead Center CAI Controlled Auto Ignition CCI Combined Combustion System CFD Computational Fluid Dynamics CI Compression Ignition CJ Chapman Jouget DFT Discrete Fourier Transform DI Direct Injection DME Dimethyl Ether EGR Exhaust Gas Recirculation FIR Finite Impulse Response FFT Fast Fourier Transform GCI Gasoline Compression Ignition HCCI Homogenous Charge Compression Ignition HTR High Temperature Reactions IIR Infinite Impulse Response IMEP Indicated Mean Effective Pressure LHV Lower Heating Value LTR Low Temperature Reactions NTSEL National Traffic Safety and Environment Laboratories PCCI Premixed Charge Compression Ignition PCI Premixed Compression Ignition RMS Root Mean Square SI Spark Ignition SPL Sound Pressure Level TDC Top Dead Center ZND Zel´dovich Neumann Döring - 8 - - 3 Résumé This thesis is based on experimental and numerical studies on the use of dimethyl ether (DME) in the homogeneous charge compression ignition (HCCI) combustion process. The first paper in this thesis was published in 2007 and describes HCCI combustion of pure DME in a small diesel engine. The tests were designed to investigate the effect of engine speed, compression ratio and equivalence ratio on the combustion timing and the engine performance. It was found that the required compression ratio depended on the equivalence ratio used. A lower equivalence ratio requires a higher compression ratio before the fuel is burned completely, due to lower in-cylinder temperatures and lower reaction rates. The study provided some insight in the importance of operating at the correct compression ratio, as well as the operational limitations and emission characteristics of HCCI combustion. HCCI combustion process is governed mainly by chemical kinetics. To understand the combustion process therefore requires detailed knowledge of the dominating reaction paths in lean premixed combustion of DME. The reactions were studied by running simulations of HCCI combustion in CHEMKIN II [1] with a detailed reaction mechanism for DME developed at Lawrence Livermore National Laboratory in 2004 [2]. The dominating reactions paths were then identified and used to create a simple reaction mechanism containing 55 reactions only. It contains just enough reactions to successfully predict ignition as well as low and high temperature reactions with reasonable similarity to the original mechanism. By reducing the mechanism to its essential reactions it becomes more useful to CFD models of HCCI combustion. The number of elementary reactions has a great influence on computational demands and computing time, so the use of a simple mechanism greatly reduces both. Reaction paths for methanol and methane were included amongst the elementary reactions, since these two fuels are commonly used to control the radical behavior in the initial phase of combustion and hence the combustion phasing of the fuel in an engine, as well as enabling an increase in engine power. The use of methanol for combustion phasing control was tested successfully in a large diesel engine with common rail, in which the piston bowls were widened to give a compression ratio of 14.5. This compression ratio still allows DI CI operation with DME, but requires a substantial combustion delay in HCCI operation with DME to achieve post TDC combustion. By adding methanol to the inlet port during HCCI combustion of DME, the engine reached 50 percent of its full DI CI load capability without engine knock at 1000 rpm and somewhat less at 1800 rpm. The engine also had EGR capability which was used to demonstrate the effect on HCCI combustion phasing of increasing EGR ratios.
Details
-
File Typepdf
-
Upload Time-
-
Content LanguagesEnglish
-
Upload UserAnonymous/Not logged-in
-
File Pages147 Page
-
File Size-