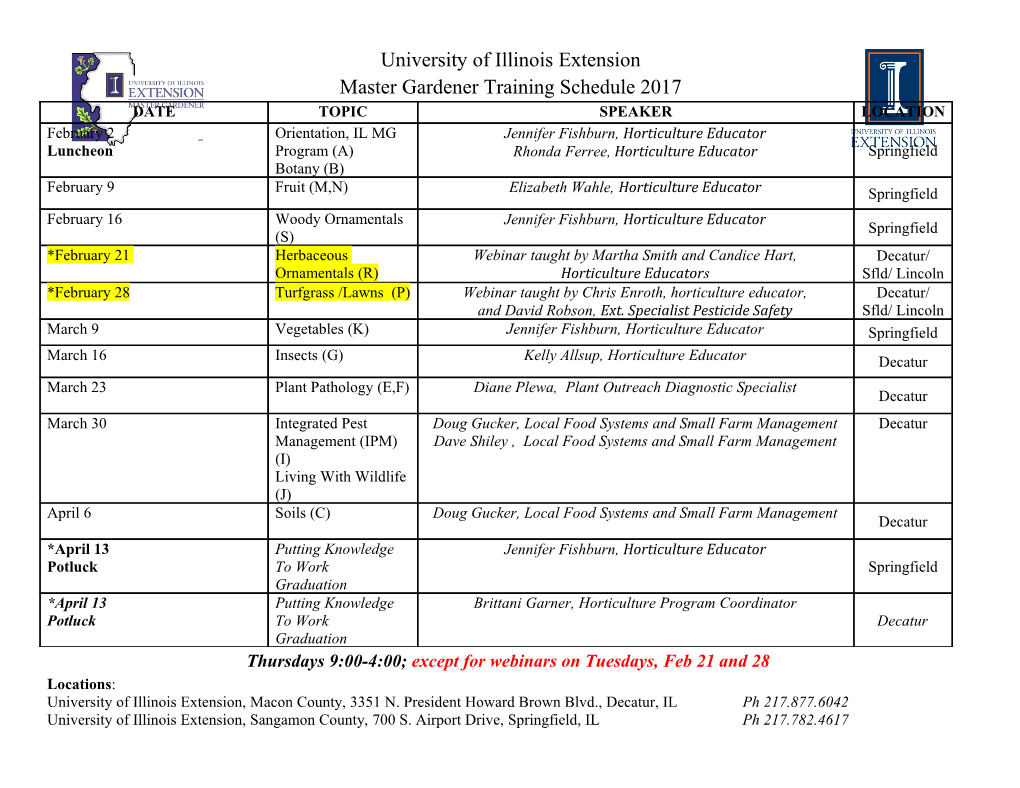
Chapter 7 Assessing and controlling the risk of cyanobacterial blooms Nutrient loads from the catchment Ingrid Chorus and Matthias Zessner CONTENTS Introduction and general considerations 434 7.1 Determining targets for nutrient concentrations in the waterbody 437 7.2 Determining critical nutrient loads to the waterbody 440 7.3 Identifying key nutrient sources and pathways causing loads 443 7.3.1 Background information 443 7.3.2 Identifying nutrient sources and pathways 446 7.3.3 Nutrient loads from wastewater, stormwater and commercial wastewater 451 7.3.3.1 Sources 452 7.3.3.2 Pathways 453 7.3.3.3 What to look for when compiling an inventory of loads from sewage, stormwater and commercial wastewater sources 454 7.3.4 Nutrient loads from agriculture and other fertilised areas 456 7.3.4.1 Sources 456 7.3.4.2 Pathways 457 7.3.4.3 What to look for when compiling an inventory of loads from agricultural activities 459 7.3.5 Nutrient loads from aquaculture and fisheries 463 7.3.5.1 What to look for when including aquaculture and fisheries in the inventory of activities causing nutrient loads 463 7.4 Approaches to quantifying the relevance of sources and pathways 465 7.4.1 Tier 1: Assessment using emission factors 468 7.4.1.1 Municipalities 468 7.4.1.2 Agriculture 470 7.4.2 Tier 2: Assessment using the Riverine Load Approach 472 7.4.2.1 Flow normalisation to avoid misinterpretation of causalities 474 7.4.2.2 Estimation of diffuse loads 474 7.4.3 Tier 3: Pathway-Oriented Approach 476 433 434 Toxic Cyanobacteria in Water 7.4.4 Tier 4: The Source-Oriented Approach 480 7.5 Managing nutrient loads 482 7.5.1 Measures to control nutrient loads from sewage, stormwater and commercial wastewater 486 7.5.1.1 Operational monitoring for control measures in wastewater management 489 7.5.1.2 Validation of control measures in sewage and stormwater management 490 7.5.2 Measures to control nutrient loads from agriculture and other fertilised areas 490 7.5.2.1 Operational monitoring of control measures in agriculture and land use involving fertilisation 494 7.5.2.2 Validation of control measures in agriculture and for land use involving fertilisation 494 7.5.3 Measures to control nutrient loads from aquaculture and fisheries 495 7.5.3.1 Operational monitoring of control measures in aquaculture and fisheries 495 7.6 Including climate change scenarios when planning measures 497 References 498 INTRODUCTION AND GENERAL CONSIDERATIONS As discussed in Chapter 5, cyanobacterial blooms in surface waters are most effectively and sustainably controlled by limiting nutrient concentra- tions in the waterbody, and this requires sufficiently limiting the nutrient loads that it receives from its catchment (for terminology, see Box 7.1). These loads enter a waterbody from point sources such as discharges and sewage outfalls and from nonpoint sources (also termed “diffuse sources”) such as surface run-off or drainage from fields. In some cases, inflow of groundwater may also carry significant nutrient loads. Furthermore, sediments may release nutrients, particularly phosphorus (P), into the waterbody. These releases are termed “internal loads”, and they delay the decline of concentrations in the water after the external load has been reduced. However, already Vollenweider and Kerekes (1980) showed that in many cases, sediments are – on an annual scale – a sink rather than a source for phosphorus; thus, if the external load reduction is effective and water exchange rates are sufficiently high, the sediments will become a sink again, typically several years after load reduction. While such time spans may be of concern, particularly if a rapid remediation is necessary or water exchange rates are low, the first step for the target of reducing nutrient concentrations in the waterbody is to reduce the external load; otherwise, measures to reduce the internal load have little chance of being sustainably effective. Assessing the role of sediment nutrient release and 7 Managing nutrient loads 435 BOX 7.1: TERMINOLOGY A catchment is the entire land area from which rain, snowmelt or g roundwater drain into a waterbody, typically delineated by the crests of the hills or mountains that form water divides. Synonyms include “watershed”, “river basin” and “drainage area”. Catchments span a very wide range from fairly small, for example, for the close surroundings of hydrologically isolated ponds, to a continental scale for large rivers. Point sources release nutrients to a waterbody at a single localised point of discharge, such as a sewage outfall. Diffuse sources, also termed “nonpoint sources”, are many smaller or scattered sources from which nutrients may be released to a waterbody, for example, from the land surface through rainwater runoff, through groundwa- ter or from scattered rural dwellings. The combined impact of diffuse sources on the waterbody may be significant. Riverine loads are the mass of a contaminant transported per unit of time, typically expressed as kg or tons per year. The nutrient load in the river reflects the sum of inputs upstream of the monitoring point at which these loads are calculated minus the possible retention in the river sediment. As such, these loads provide a first check: the sum of inputs from individual and separate sources should broadly equate to the total riverine load if retention is neglected for very rough estimation. More detailed investigations include retention. Riparian buffer strips are the areas around a waterbody of about 10–30 m width or more, covered with dense vegetation which can effectively intercept surface run-off carrying phosphorus-rich soil eroded from arable land and pastures. Tile drainage is a term used for draining land that would otherwise be too saturated with water for crops to grow. The term derives from installing drainage in a grid pattern covering the otherwise too moist field. options for controlling it are discussed in section 8.6. This chapter focuses on assessing and managing external nutrient loads to a waterbody. As outlined in Figure 7.1, the first step for this purpose is to estimate the maximum nutrient concentration in the waterbody that can be tolerated to effectively control (toxic) cyanobacterial blooms (section 7.1) and the corresponding load to the waterbody that may be tolerated to avoid exceed- ance of this target concentration (section 7.2). The next steps are to identify the main pathways and sources of nutrients (section 7.3) and to estimate the respective loads they contribute (section 7.4). The approach to esti- mating these loads may range from qualitative expert judgement (includ- ing that of local stakeholders) to quantitative load modelling (see tiers in 436 Toxic Cyanobacteria in Water Determine the target nutrient concentration in the waterbody to effectively control cyanobacteria → 7.1 Estimate the critical nutrient load to reach this target load → 7.2 Identify potential pathways and sources of nutrients → 7.3 Assess the respective loads they contribute to the total load reaching the water-body → 7.4 Identify and implement measures to control nutrient loads, including climate change scenarios → 7.5 & 7.6 Figure 7.1 Steps in the selection of measures for controlling nutrient loads from the catchment. section 7.4), depending on the available information. Once the loads from key pathways and sources are clear, the next step is to identify the most promising and most cost-effective measures to control them, to implement these measures, and to ensure they are operating effectively (section 7.5). After the implementation of measures, it is important to monitor whether they are taking effect as planned. This involves going back to assessing the nutrient load in order to validate that it has been sufficiently reduced, and it involves monitoring the nutrient concentration in the waterbody. Implementing effective measures to control nutrient loads takes time, often several years, and particularly for P, it takes further time for con- centrations in the waterbody to decline and for biota to respond to lower concentrations: Jeppesen et al. (2005) reviewed data on the responses to a nutrient load reduction of 35 lakes and found that at least 3 retention times (i.e., exchanges of the lake’s volume) were necessary to dilute 95% of the excess P out of the waterbody and <10–15 years for total phosphorus (TP) to reach a new equilibrium between water and sediment; deep lakes tend to take longer. Although time scales of years or even decades may seem prohibitive, in the longer term controlling cyanobacterial blooms through keeping the nutrient load sufficiently low is the most sustainable approach, often rendering further (usually costly and continuously necessary) mea- sures within the waterbody (discussed in Chapter 8) unnecessary. 7 Managing nutrient loads 437 The guidance given in this chapter for assessing and controlling nutrient loads from catchments to waterbodies is valid independently of the size of the catchment. Estimating nutrient loads from large catchments with a range of possible nutrient sources can be particularly challenging. For rivers crossing municipal, state or national borders, planning and man- agement require collaboration across jurisdictions, and for transnational basins, international commissions have proven useful. For example, the Water Framework Directive of the European Union requires transboundary river basin management plans. Nutrient loads are more readily assessed and controlled for smaller, more readily controllable catchments, for example, those of reservoirs in middle-range mountains. For further information and guidance on managing waterbodies and their catchments, readers are referred to the WHO guidebook Protecting Surface Water for Health (Rickert et al., 2016). 7.1 DETERMINING TARGETS FOR NUTRIENT CONCENTRATIONS IN THE WATERBODY Which nutrient to address – P or both N and P – is a key question for plan- ning measures to reduce the maximum possible amount of phytoplankton biomass – and thus of cyanobacteria – in a given waterbody.
Details
-
File Typepdf
-
Upload Time-
-
Content LanguagesEnglish
-
Upload UserAnonymous/Not logged-in
-
File Pages71 Page
-
File Size-