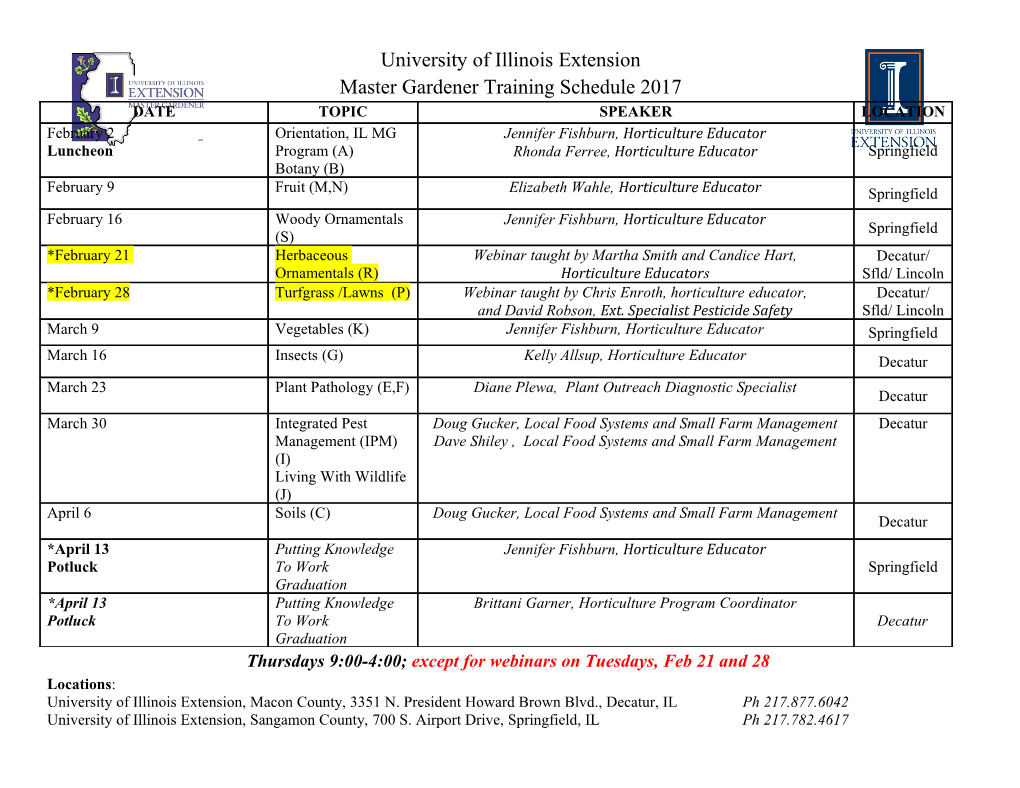
CARNEGIE MELLON UNIVERSITY CARNEGIE INSTITUTE OF TECHNOLOGY THESIS SUBMITTED IN PARTIAL FULFILLMENT OF THE REQUIREMENTS FOR THE DEGREE OF DOCTOR OF PHILOSOPHY ELECTRIC POWER SYSTEMS UNDER STRESS: AN EVALUATION OF CENTRALIZED VERSUS DISTRIBUTED SYSTEM ARCHITECTURES by Hisham Zerriffi ACCEPTED BY THE DEPARTMENT OF ENGINEERING AND PUBLIC POLICY Major Professor Date Major Professor Date Department Head Date APPROVED BY THE COLLEGE COUNCIL Dean Date Carnegie Mellon University Electric Power Systems Under Stress: An Evaluation Of Centralized Versus Distributed System Architectures A DISSERTATION SUBMITTED TO THE GRADUATE SCHOOL IN PARTIAL FULFILLMENT OF THE REQUIREMENTS for the degree of DOCTOR OF PHILOSOPHY in ENGINEERING AND PUBLIC POLICY by Hisham Zerriffi Pittsburgh, Pennsylvania September, 2004 © Copyright 2004 by Hisham Zerriffi. All rights reserved. Hisham Zerriffi ii Electric Power Systems Under Stress In memory of my grandfather, Dr. Henri M. Yaker, whose great intellect, diverse academic and professional pursuits, and love of life and family have been an inspiration. I only hope to live up to his example. iii Hisham Zerriffi iv Electric Power Systems Under Stress Abstract It is well recognized that electric power systems do not always perform perfectly and that maintaining reliability of supply is one of the important tasks for power system planners. However, there are circumstances under which power systems can face persistent stresses or have the possibility of being under high stress conditions. These stresses arise from and affect both the technical systems designed to generate and deliver electricity, as well as the commercial and political organizations designed to undertake those tasks and to govern these activities. The issue of electric power systems under persistent and high stress conditions and possible changes to electric power systems to deal with this issue is the subject of this dissertation. The stresses considered here are not the single event type of disruptions that occur as a result of a hurricane or other extreme weather event or the large blackouts that result from a particular set of circumstances. Instead the focus is on conditions that cause systematic and long-term performance degradation of the system. Electric power systems have been and will continue to be challenged by a number of stress conditions that can come from a variety of sources. Extreme weather has long been recognized as a contingency that must be planned for as best as possible and then mitigated against when it occurs. However, there are a number of other stressors that could have effects that rival that of extreme weather events (or could be even worse). Such stressors can have very different characteristics than extreme weather events (e.g. in the scope and nature of the stress or the persistence of the stress) rendering some of the solutions developed for extreme weather events less suitable for such contingencies. Example of such a stress include underinvestment in infrastructure (e.g. transmission), v Hisham Zerriffi lack of access to spare parts or trained personnel and the set of problems created for electric power systems during conflict situations. The particular stress conditions faced by an electric power system will depend heavily on context and will likely be different for industrialized versus industrializing countries. It has long been recognized that stresses such as conflict and war can have a large impact on electric power systems. There have even been arguments made regarding how changes in the system architecture from a centralized to a distributed system can aid in mitigating the impacts of such stresses. However, there have been few systematic analyses of the problem. The contribution of this research has been primarily in three areas: • The systematic characterization of stresses on electricity infrastructure • The development of a method to analyze stress on electric power systems (particularly the application of a stress adjustment factor and the creation of non- coincident demand) • The quantification of the impacts of making a large-scale change to a distributed system architecture and the reliability impacts of changing to such a system under stress conditions, including the impacts of fuel supply. Some of the issues related to having a system based upon a significant level of distributed generation that have been explored include the reliability of different mixes of centralized and distributed generation technologies, the impact of load coincidence on reliability and needed DG investment, and changes in the usage of the high voltage transmission network. vi Electric Power Systems Under Stress As expected, a model that compares a hypothetical centralized system with a completely distributed system demonstrates that the distributed system has two reliability advantages. First, under normal operating conditions it is possible to reduce drastically, or almost eliminate, the reserve margin in the system while at the same time improving the adequacy of the system to provide real power (as measured in MWh/year not supplied). Second, under stress conditions the reliability advantages of most distributed systems are demonstrably higher than a centralized system of standard design. Most notably, as stress levels are increased, the loss of energy expectation does not rise as rapidly for the distributed systems as for the centralized system. These are reliability advantages for the whole system. Furthermore, under normal conditions, the decrease in required capacity and the use of waste heat for co-generation make the distributed system competitive with a centralized system in terms of levelized cost of electricity supply. Under stress conditions, the economic losses suffered by various sectors of the economy become increasingly significant. The improved performance of the distributed system under stress translates directly into economic benefits. The model was also extended to include the likely supporting natural gas infrastructure for currently competitive DG technologies in order to determine the impact of dependence upon that infrastructure on the reliability of the system. It was found that under normal operating circumstances, due to the high reliability of natural gas systems, there is a negligible impact on the reliability of the power system. In order for stress on the natural gas system to have a significant impact vii Hisham Zerriffi on electricity provision, the stress level has to result in unavailabilities that are hundreds of times higher than the baseline reliability of the natural gas system. This might occur, for example, if the system is above ground and easily targeted. While it is useful to think of the two ends of the centralized/distributed spectrum, as was done in the initial engineering economic modeling (see Chapters 3-5) and in the natural gas network model, the reality is that any power system will be a mix of distributed and centralized generating technologies. In Chapter 6 a number of mixed system topologies were evaluated using the engineering-economic model. The model was re-run with various strategies for replacing centralized with distributed generation. In each case, the amount of centralized generation removed from the system was replaced with differing amounts of distributed generation. The results of these model runs were compared with model runs for the purely centralized and purely distributed systems. The results indicate that three inter-related factors are critically important in determining the relative performance of the different mixed systems. First is the relative size of the centralized units being replaced. This is due to the fact that the impact of any particular generator failing is dependent upon its size. Replacing larger units results in a system composed entirely of distributed and relatively small or medium sized centralized units and a decreased dependency on a few large units. Second are the relative reliability characteristics of the centralized units being replaced. Replacing highly reliable centralized units with less reliable (though more numerous) distributed units leads to no change. The third is the degree of distributed generation penetration and resulting reserve capacity. At high penetration rates (e.g. on the order of 40%) it is possible to maintain or viii Electric Power Systems Under Stress even significantly improve system performance while at the same time reducing overall generation capacity and essentially eliminating the reserve capacity. The result is that the needed reserve margin is not a fixed number but highly dependent on the mix of technologies and the degree of DG penetration. The reliability of the system is not simply a function of the generation and transmission of electricity. The other side of the equation is the demand for electricity itself. Specifically, reliability is determined by comparing the energy that can be delivered in any given time period with the demand for that time period. In order to assess some of the impacts of load non-coincidence on reliability and on power sharing between micro- grids, a method was established to systematically vary the loads at different customer load buses. The result is demand profiles that are not artificially set to be coincident and therefore, there are greater opportunities for sharing power than might be indicated in a model that does not include differentiated non-coincident loads. The results show the inter-relationship between installed capacity at the micro-grids,
Details
-
File Typepdf
-
Upload Time-
-
Content LanguagesEnglish
-
Upload UserAnonymous/Not logged-in
-
File Pages280 Page
-
File Size-