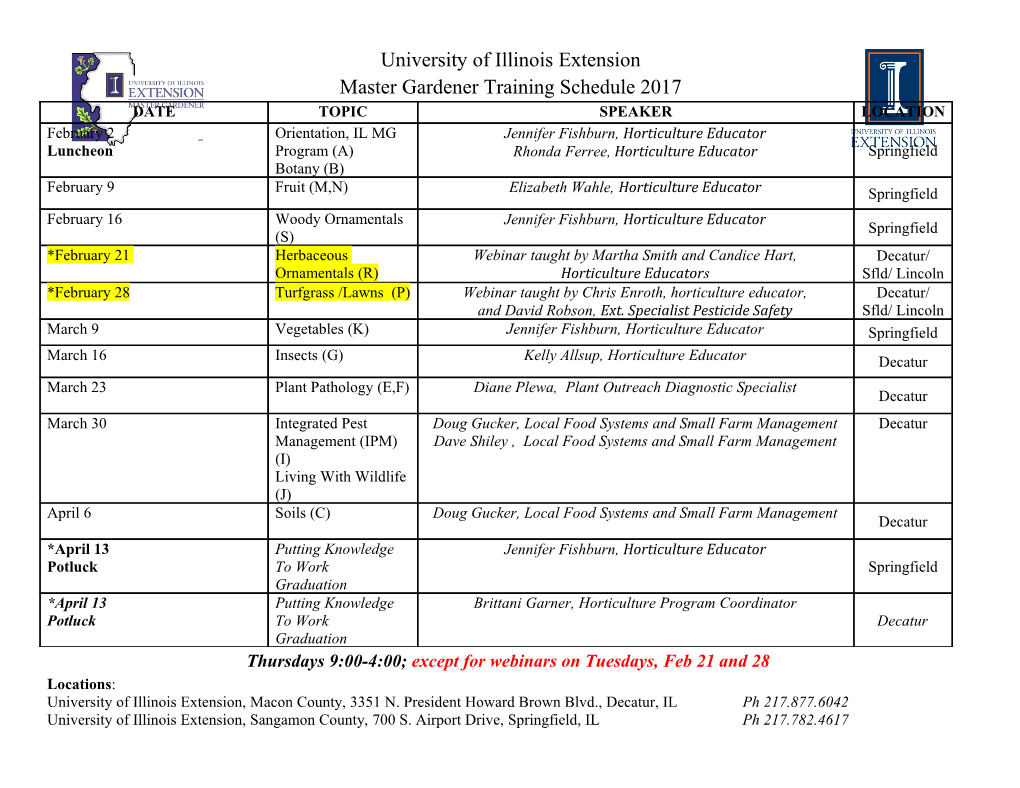
RESEARCH ARTICLE Conserved RNA-binding specificity of polycomb repressive complex 2 is achieved by dispersed amino acid patches in EZH2 Yicheng Long1, Ben Bolanos2†, Lihu Gong3,4†, Wei Liu2, Karen J Goodrich1, Xin Yang3,4, Siming Chen3,4, Anne R Gooding1, Karen A Maegley5, Ketan S Gajiwala2, Alexei Brooun2*, Thomas R Cech1*, Xin Liu3,4* 1Department of Chemistry and Biochemistry, BioFrontiers Institute, Howard Hughes Medical Institute, University of Colorado Boulder, Boulder, United States; 2Worldwide Medicinal Chemistry, Worldwide Research and Development, Pfizer Inc., San Diego, United States; 3Cecil H. and Ida Green Center for Reproductive Biology Sciences, Division of Basic Research, Department of Obstetrics and Gynecology, UT Southwestern Medical Center, Dallas, United States; 4Department of Biophysics, UT Southwestern Medical Center, Dallas, United States; 5Oncology Research Unit, Worldwide Research and Development, Pfizer Inc., San Diego, United States Abstract Polycomb repressive complex 2 (PRC2) is a key chromatin modifier responsible for methylation of lysine 27 in histone H3. PRC2 has been shown to interact with thousands of RNA *For correspondence: species in vivo, but understanding the physiological function of RNA binding has been hampered [email protected] (AB); by the lack of separation-of-function mutants. Here, we use comprehensive mutagenesis and [email protected] hydrogen deuterium exchange mass spectrometry (HDX-MS) to identify critical residues for RNA (TRC); interaction in PRC2 core complexes from Homo sapiens and Chaetomium thermophilum, for which [email protected] (XL) crystal structures are known. Preferential binding of G-quadruplex RNA is conserved, surprisingly † These authors contributed using different protein elements. Key RNA-binding residues are spread out along the surface of equally to this work EZH2, with other subunits including EED also contributing, and missense mutations of some of Competing interest: See these residues have been found in cancer patients. The unusual nature of this protein-RNA page 20 interaction provides a paradigm for other epigenetic modifiers that bind RNA without canonical Funding: See page 20 RNA-binding motifs. DOI: https://doi.org/10.7554/eLife.31558.001 Received: 25 August 2017 Accepted: 09 November 2017 Published: 29 November 2017 Reviewing editor: Ali Introduction Shilatifard, Northwestern Polycomb Repressive Complex 2 (PRC2) is essential for epigenetic silencing of gene expression dur- University, United States ing embryonic development and disease pathogenesis (Schuettengruber and Cavalli, 2009; Copyright Long et al. This Margueron and Reinberg, 2011; Helin and Dhanak, 2013). PRC2 specifically catalyzes mono-, di- article is distributed under the and tri-methylation of lysine 27 on histone H3 (H3 K27), which is a hallmark for repressed chromatin terms of the Creative Commons and provides a chromatin structure that is repressive to transcription (Cao et al., 2002; Attribution License, which Czermin et al., 2002; Mu¨ller et al., 2002; Yuan et al., 2012). The PRC2 core complex contains four permits unrestricted use and subunits: (1) EZH2 (enhancer of zeste homolog 2) or its closely related homolog EZH1, (2) SUZ12 redistribution provided that the original author and source are (suppressor of zeste 12), (3) EED (embryonic ectoderm development) and (4) RBBP4 (retinoblastoma credited. binding protein 4) (Margueron and Reinberg, 2011). The complex can include additional Long et al. eLife 2017;6:e31558. DOI: https://doi.org/10.7554/eLife.31558 1 of 23 Research article Biochemistry subcomponents such as AEBP2 (adipocyte enhancer-binding protein 2). EZH2 contains the enzy- matic SET (Su[var]3–9, enhancer of zeste, trithorax) domain, which catalyzes the transfer of methyl groups from S-adenosylmethionine (SAM) to H3 K27 (Rea et al., 2000; Dillon et al., 2005; Joshi et al., 2008). How human PRC2 is recruited to specific sites and regulated on chromatin in mammals is an important unanswered question. Multiple factors including chromatin marks, DNA sequence ele- ments and other DNA-binding proteins (e.g. JARID2) have been suggested to recruit PRC2 to its genomic targets (Lee et al., 2006; Martin et al., 2006; Ku et al., 2008; Margueron et al., 2009; Peng et al., 2009; Schuettengruber and Cavalli, 2009; Shen et al., 2009; Sing et al., 2009; Li et al., 2010; Pasini et al., 2010; Woo et al., 2010; Xu et al., 2010; Cuddapah et al., 2012; Yuan et al., 2012; Riising et al., 2014). Although long noncoding RNAs (lncRNA) have been sug- gested to act as guides for recruitment of PRC2 to chromatin in cis or in trans (Fitzpatrick et al., 2002; Mak et al., 2002; Plath et al., 2003; Silva et al., 2003; Rinn et al., 2007; Pandey et al., 2008; Zhao et al., 2008; Kanhere et al., 2010; Heo and Sung, 2011), recent studies have empha- sized the ability of RNA to inhibit PRC2 methylation of histones (Cifuentes-Rojas et al., 2014; Kaneko et al., 2014; Beltran et al., 2016; Wang et al., 2017a). Numerous RNAs were identified to interact with PRC2 in vivo using RNA immunoprecipitation approaches (Zhao et al., 2010; G Hendrickson et al., 2016). PRC2-binding RNAs include mRNAs as well as lncRNAs such as Kcnqtlot1, ANRASSF1, and COLDAIR (Rinn et al., 2007; Pandey et al., 2008; Redrup et al., 2009; Heo and Sung, 2011; Beckedorff et al., 2013). PRC2 binds RNA promiscuously in vitro and in vivo (Davidovich et al., 2013, 2015), meaning that it binds many natural RNAs with affinities within the same order of magnitude. This promiscuous binding can be explained by PRC2 recognizing short tracts of G’s and G-quadruplexes, small motifs which are ubiquitous in the transcriptome (Wang et al., 2017b). What elements within PRC2 bind RNA has been unclear, especially since none of the core PRC2 subunits has an RNA recognition motif (RRM). An unstructured region (residues 342–368) of mouse EZH2 that has been proposed to bind RNA (Kaneko et al., 2010) is not phylogenetically conserved (Jiao and Liu, 2015), and more important elements for RNA interaction are now apparent (see below). Separation of the RNA-binding role of PRC2 from its other activities – formation of the cata- lytically competent complex, recognition of histone tails, methyltransferase activity and interactions with accessory proteins – would greatly help to identify any PRC2 recruitment events that are depen- dent on RNA binding and to characterize RNA-mediated epigenetic silencing or inhibition of silencing. A 21 A˚ electron microscopy structure of the human PRC2 complex shed light on its molecular architecture and subunit organization (Ciferri et al., 2012), and recently three high-resolution crystal structures of the minimal catalytic core of PRC2 (EZH2, EED and Suz12-VEFS) were determined. These include a complex from the fungus Chaetomium thermophilum (ctPRC2) (Jiao and Liu, 2015), a chimeric ac/hsPRC2 containing EZH2 from the American chameleon (Anolis carolinensis) and the remaining subunits from H. sapiens (Brooun et al., 2016) and an all-human PRC2 complex (Justin et al., 2016). Surprisingly, no canonical RNA-binding motif can be identified in these structures. In this study, we find that the specificity of PRC2 for G-rich RNA and G-quadruplex RNA, previ- ously shown for hsPRC2 (Wang et al., 2017b), is shared with ctPRC2, suggesting that the PRC2- RNA specificity could be conserved over a vast evolutionary distance. Surprisingly, while EZH2 emerges as the critical RNA-binding subunit for both ctPRC2 and hsPRC2, different sets of residues are involved. The manner in which RNA-binding elements are dispersed across the surface of PRC2 is unanticipated, and may portend similarly complex binding surfaces in other histone modification complexes and DNA methyltransferases that bind RNA. Results The active fungal ctPRC2 complex exemplifies similar RNA-binding specificity as human PRC2 The PRC2-RNA interaction has been exclusively studied in mammals, and expanding such knowl- edge to additional organisms would help to understand the biological importance of this interaction Long et al. eLife 2017;6:e31558. DOI: https://doi.org/10.7554/eLife.31558 2 of 23 Research article Biochemistry through evolution. We therefore characterized the RNA-binding specificity of a crystallized PRC2 complex (ctPRC2) from a thermophilic fungus (Jiao and Liu, 2015). We first measured the binding app affinity (illustrated by Kd ) of purified ctPRC2 with a variety of synthetic homopolymeric 40-mer RNAs. ctPRC2 had specificity for poly (G) RNA, while having the lowest affinity for poly (A) RNA (Figure 1A). Second, ctPRC2 preferred binding to RNA sequences capable of folding into G-quadru- plexes ((GGAA)10, (G4A4)4 and G40)(Figure 1B and C). For example, (GGAA)10 and (GA)20 RNA A B ct PRC2 ct PRC2 (GGAA) 10 RNA - - KCl buffer app poly (A) Kd = 19 ± 8 nM RNA app >> 5µM Kd LiCl buffer app poly (C) RNA Kd = 1300 ± 78 nM K app = 1100 ± 150 nM d ct PRC2 C - (GA) 20 RNA poly (U) RNA KCl buffer app Kd = 1400 ± 160 nM app Kd = 1100 ± 21 nM poly (G) LiCl buffer app RNA Kd = 1400 ± 84 nM app Kd = 91 ± 8 nM D Fold Fold change of K app in K+ K app in Li + change of K app in Li + / RNA ! sequence ! d d d K app + K app +! (nM)! (nM)! d in Li / d in K for K app +! hs d in K PRC2 * ! poly (G) ! G40 ! 91 ± 8! 360 ± 64 ! 4! 7! poly (C) ! C40 ! 1100 ± 150 ! 980 ± 17 ! 0.9 ! 0.6 ! poly (U) ! U40 ! 1400 ± 160 ! 1200 ± 78 ! 0.9 ! 1! poly (A) ! A40 ! >5000 ! >5000 ! N/A ! N/A ! (GA) 20 ! (GA) 20 ! 1100 ± 21 ! 1400 ± 84 ! 1.3 ! 0.8 ! (GGAA) 10 ! (GGAA) 10 ! 19 ± 8! 1300 ± 78 ! 68 ! 40 ! (G4A4)4 ! A6G4A4G4A4 89 ± 14 ! 1800 ± 100 ! 20 ! 7! G4A4G4A6! (G4A4)3 ! A6G4A4G4A4 2000 ± 100 ! 2500 ± 290 ! 1.3 ! 2! G4A14 ! Figure 1. ctPRC2 prefers binding to G-rich RNAs and G-quadruplexes, and shares RNA-binding specificity with hsPRC2.
Details
-
File Typepdf
-
Upload Time-
-
Content LanguagesEnglish
-
Upload UserAnonymous/Not logged-in
-
File Pages23 Page
-
File Size-