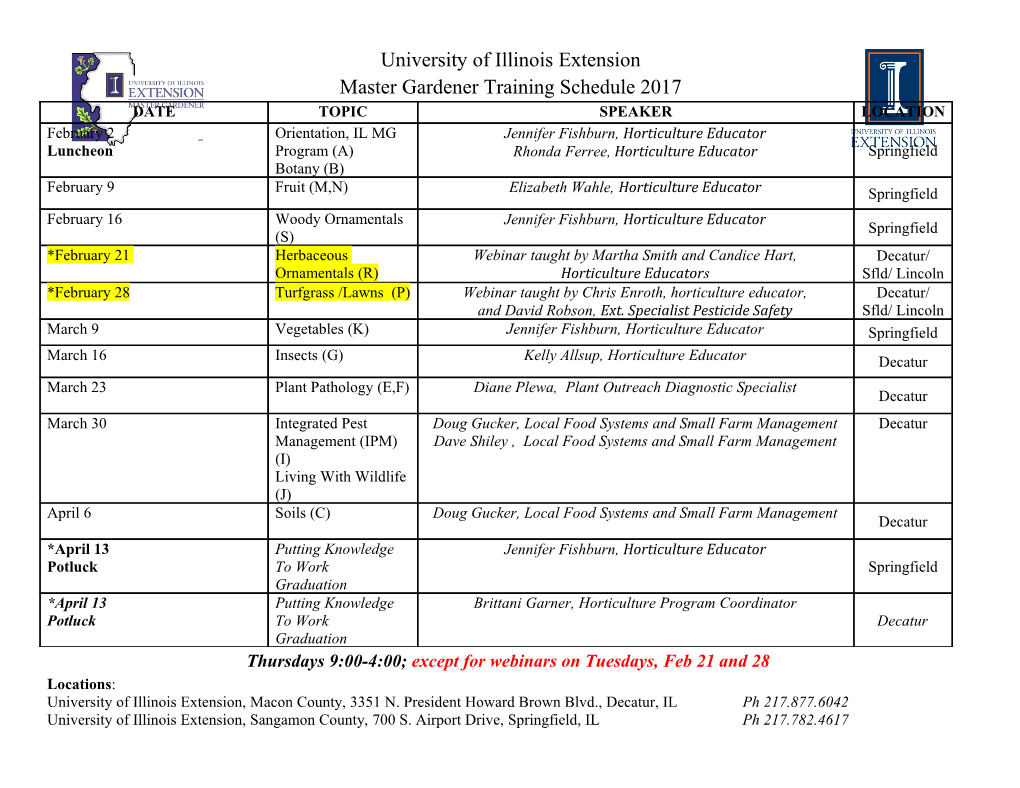
FINITE DIMENSIONAL GRADING OF THE VIRASORO ALGEBRA RUBEN´ A. HIDALGO1), IRINA MARKINA2), AND ALEXANDER VASIL’EV2) Abstract. The Virasoro algebra is a central extension of the Witt algebra, the complexified Lie algebra of the sense preserving diffeomorphism group of the circle Diff S1. It appears in Quantum Field Theories as an infinite dimen- sional algebra generated by the coefficients of the Laurent expansion of the analytic component of the momentum-energy tensor, Virasoro generators. The background for the construction of the theory of unitary representations of Diff S1 is found in the study of Kirillov’s manifold Diff S1/S1. It possesses a natural K¨ahlerian embedding into the universal Teichm¨uller space with the projection into the moduli space realized as the infinite dimensional body of the coefficients of univalent quasiconformally extendable functions. The dif- ferential of this embedding leads to an analytic representation of the Virasoro algebra based on Kirillov’s operators. In this paper we overview several inter- esting connections between the Virasoro algebra, Teichm¨uller theory, L¨owner representation of univalent functions, and propose a finite dimensional grad- ing of the Virasoro algebra such that the grades form a hierarchy of finite dimensional algebras which, in their turn, are the first integrals of Liouville partially integrable systems for coefficients of univalent functions. 1. Introduction The Virasoro-Bott group vir appears in physics literature as the space of reparametrization of a closed string. It may be represented as the central ex- tension of the infinite dimensional Lie-Fr´echet group of sense preserving diffeo- morphisms of the unit circle. The corresponding Virasoro algebra Vir is realized as the central extension of the algebra of vector fields on S1. The coadjoint or- bits of the Virasoro-Bott group are related to the unitary representation of vir as an analogue to the representation of finite dimensional compact semi-simple Lie groups given by the Borel-Weil-Bott theorem, see [36]. Two orbits are of particular importance because they carry the structure of infinite dimensional 1 1 1 homogeneous K¨ahlerian manifolds. They are Diff S /S and Diff S /SL2(R), both are homogeneous complex analytic Fr´echet-K¨ahler manifolds. 2000 Mathematics Subject Classification. Primary 30C50, 17B68; Secondary 53C17, 70H06. Key words and phrases. Univalent function, coefficient, Virasoro algebra, Hamiltonian sys- tem, Teichm¨uller space, L¨owner equation. 1)Supported by FONDECYT (Chile) #1070271, and by UTFSM (Chile) #12.05.21. 2)Supported by the Norwegian Research Council NFR 177355/V30, by the European Sci- ence Foundation Networking Programme HCAA. 1 2 R.A.HIDALGO,I.MARKINA,ANDA.VASIL’EV We deal with the analytic representation of Diff S1/S1. Let U be the unit disk U = {z : |z| < 1}. Let S stand for the standard class of holomorphic univalent functions f : U → C normalized by ∞ n f(z)= z 1+ cnz , z ∈ U. n=1 ! X By S˜ we denote the class of functions from S smooth (C∞) on the boundary S1 of U. Given a map f ∈ S˜ we construct an adjoint univalent meromorphic map d− g(z)= d z + d + 1 + ..., 1 0 z defined in the exterior U ∗ = {z : |z| > 1} of U, and such that Cˆ \f(U)= g(U ∗). This gives the identification of Diff S1/S1 with the space of smooth contours Γ that enclose univalent domains Ω of conformal radius 1 with respect to the origin and such that ∞ 6∈ Ω, 0 ∈ Ω, see [1, 19]. Being quasicircles, the smooth contours allows us to embed Diff S1/S1 into the universal Teichm¨uller space making use of the above conformal welding, and then, to project it to the set N M ⊂ C which is the limiting set for the coefficient bodies M = limn→∞ Mn, where (1) Mn = {(c1,...,cn) : f ∈ S˜}. Then the Virasoro generators may be realized by the first order differential operators ∞ Lj = ∂j + (k + 1)ck∂j+k, j ∈ N, Xk=1 in terms of the affine coordinates of M, acting over the set of holomorphic functions, where ∂k = ∂/∂ck. A representation [1] of the Virasoro algebra into the Lie algebra of the differential operators on S˜ may be given by means of the Neretin [27] homogeneous polynomials Pk(c1,...,ck) given by the recurrence relation (2), where ∞ 12 P (c ,...,c )zn = S (z), z2 n 1 n f n=0 and X f ′′′(z) 3 f ′′(z) 2 S (z)= − f f ′(z) 2 f ′(z) is the Schwarzian derivative of a univalent function f ∈ S˜. Indeed, the polyno- mials Pn satisfy the following recurrence relations 1 2 (2) L (P )=(j + k)P − + k(k − 1)δ , P ≡ P ≡ 0, P (0) = 0. k j j k 12 j,k 0 1 j Note that Lk(Pj) ≡ 0 for k > j so we do not need to define the polynomials Pj for negative indices. From the physics view-point the Virasoro generators naturally appear as the coefficients of the Laurent mode expansion of the momentum-energy tensor in FINITEDIMENSIONALGRADING... 3 the 2-D Conformal Field Theory. The Schwarzian derivative comes into play as a defect term in the chart-change formula for this tensor (its analytic component). The Virasoro algebra Vir is an infinite dimensional vector space, moreover, the dimension of this space is uncountably infinite. It is natural to reduce Vir to a finite dimensional grading by truncating and to study the resulting finite dimensional grades with respect to standard bases. Indeed, the recurrence relation (2) does not require the complete series of operators ∂j at each step. Moreover, the limiting coefficient body M is of very complicated form although we know that its projection to Cn is bounded for any fixed n ∈ N, thanks to ∞ de Branges [8]. Note that M = limn→∞ Mn =6 ∪n=1Mn. Let us construct the truncated generators n−j (3) Lj = ∂j + (k + 1)ck∂j+k, Xk=1 for j =1,...,n, Lj = 0for j > n, defined on Mn. For each fixed n we get a finite dimensional algebra An = span(L1,..., Ln) with the same commutator relation as for A = span(L1,...,Ln,... ), and satisfying the same relations (2) for the Neretin polynomials. All these algebras are disjoint and form a kind of hierarchy with respect to n. We use here the attribution ‘hierarchy’ because it turns out that the vector fields Lj naturally appear in the hierarchies of the Hamiltonian systems for the coefficient bodies for univalent functions (see [22, 30]). These systems are partially integrable in the sense of Liouville and the vector fields Lj serve as first integrals replacing ∂j by formal variables corresponding to the velocity components of the Hamiltonian systems. We also discuss the K¨ahlerian embedding of the Virasoro-Bott group/ Vira- soro algebra into the universal Teichm¨uller space/the space of harmonic Bel- trami differentials and connections between corresponding embedding of the finite dimensional grades and Teichm¨uller spaces of Fuchsian groups. Several parts of this paper have been discussed with professor Dmitri Prokhorov to whom the authors express their gratitude. 2. CN and C∞ The infinite vector space C∞ consists of infinite sequences of elements from C such that only finitely many elements are non-vanishing. The algebraic op- erations for such sequences may be introduced in the same way as for Cn. The dimension of C∞ is countably infinite. So C∞ is a coproduct of countably many copies of C. The space CN consists of sequences with possibly infinitely many non-vanishing elements. The dimension of this space is uncountably infinite and CN is the product of countably many copies of C. The space CN is dual to C∞, but they are not isomorphic (see, e.g., [15, Section 1.3]). The Virasoro algebra topologically is uncountably infinite dimensional. 4 R.A.HIDALGO,I.MARKINA,ANDA.VASIL’EV 3. Virasoro algebra and Kirillov’s operators We denote by Diff S1 the infinite dimensional Lie-Fr´echet group of sense pre- serving diffeomorphisms of the unit circle. The associated Lie algebra is the Lie 1 d algebra VectS of right-invariant vector fields φ(θ) dθ with the vanishing mean value on S1, and with the usual Lie-Poisson bracket ′ ′ 1 [φ1,φ2]= φ1φ2 − φ1φ2, φ1,φ2 ∈ VectS , where the derivative is taken with respect to the angular variable. Fixing the trigonometric basis in VectS1, the commutator relations admit the form n − m n + m [cos nθ, cos mθ] = sin (n + m)θ + sin (n − m)θ, 2 2 m − n n + m [sin nθ, sin mθ] = sin (n + m)θ + sin (n − m)θ, 2 2 m − n n + m [sin nθ, cos mθ] = cos(n + m)θ − cos(n − m)θ. 2 2 1 1 Let VectCS be the complexified Lie algebra of VectS . The topological com- inθ d plex basis vn = −ie dθ satisfies the commutation rule of the Witt algebra [vn, vm]=(m − n)vn+m. 1 The Lie algebra VectS contains span(v1,...,vn,... ) as a dense Lie subalgebra in the Fr´echet topology. The Virasoro algebra is a unique (up to isomorphism) non-trivial central 1 1 1 extension VectCS ⊕ C of VectCS by C (or Vect S by R in the real case) given by the Gelfand-Fuchs 2-cocycle [13]: 1 2 ω(v , v )= n(n − 1)δ − , n m 12 n, m and the commutation rule becomes c (4) [v , v ] =(m − n)v + ω(v , v ), n m V ir n+m 12 n m (we put c = 1 in (2)). The above cocycle is cohomologically equivalent to the cocycle 3 ω0(vn, vm)= n δn,−m, or in the functional form, 2π 2π −i −i ω (φ ,φ )= φ′ φ′′dθ = (φ′ φ′′ − φ′′φ′ )dθ.
Details
-
File Typepdf
-
Upload Time-
-
Content LanguagesEnglish
-
Upload UserAnonymous/Not logged-in
-
File Pages16 Page
-
File Size-