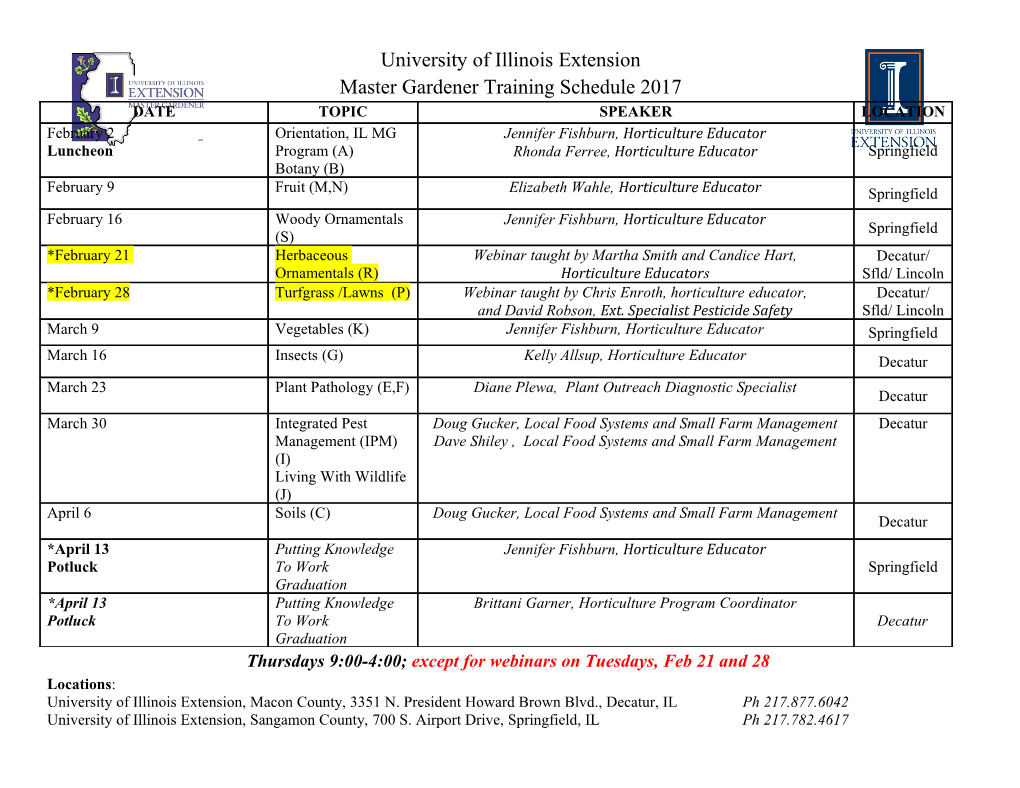
Dissecting Dopamine D2 Receptor Signaling Prashant Donthamsetti Submitted in partial fulfillment of the requirements for the degree of Doctor of Philosophy in the Graduate School of Arts and Sciences COLUMBIA UNIVERSITY 2015 © 2015 Prashant Donthamsetti All rights reserved ABSTRACT Dissecting Dopamine D2 Receptor Signaling Prashant Donthamsetti Dopamine D2 receptor (D2R) is a G protein-coupled receptor (GPCR) that activates G protein and arrestin signaling molecules. D2R antagonism has been a hallmark of antipsychotic medications for more than half a century. However, this drug-class is associated with substantial side effects that decrease quality of life and medication compliance. The development of novel antipsychotic medications with superior therapeutic and side effect profiles has been hampered in part due to a poor understanding of the specific D2R populations and downstream signaling molecules that must be blocked to confer therapeutic efficacy. It has been proposed that antipsychotic medications confer their effects through the blockade of arrestin but not G protein signaling downstream of D2R, and thus substantial efforts have gone towards the development of ligands that selectively block arrestin signaling. However, this approach suffers from several major limitations, namely that blockade of G protein signaling may also be important in conferring antipsychotic effects. Moreover, currently available pharmacological and genetic tools that have been used to probe G protein and arrestin signaling downstream of D2R in vivo suffer from on- and off-target effects that add substantial confounds to our understanding of these processes. Herein, we describe the development of several tools that can be used to probe G protein and arrestin-mediated processes in vivo with high specificity, as well as mechanisms by which these processes are activated. Table of contents List of Figures ii Acknowledgements iv Dedication v CHAPTER 1. Introduction 1 CHAPTER 2. The development of genetic tools to probe D2R-mediated arrestin signaling in vivo 25 CHAPTER 3. The development of genetic tools to probe D2R-mediated G protein signaling in vivo 44 CHAPTER 4. Towards understanding functional selectivity between G protein-dependent pathways downstream of D2R 82 CHAPTER 5. Summary and Conclusions 107 Materials and Methods 110 References 134 Appendix 150 i List of Figures Figure 1-1. Major dopaminergic pathways within the CNS. 2 Figure 1-2. Basal ganglia circuitry. 3 Figure 1-3. The crystal structure of the dopamine D3R with eticlopride. 5 Figure 1-4. Pharmacological agents vary in their functional properties. 6 Figure 1-5. G protein activation cycle. 7 Figure 1-6. DAR G protein signaling. 8 Figure 1-7. D2R and arrestin. 9 Figure 1-8. The DA synapse. D2R is expressed both pre- and postsynaptically within a DA synapse. 11 Figure 1-9. Classical pharmacological agents versus functional selective ligands. 15 Figure 1-10. G protein versus arrestin bias. 16 Figure 1-11. Characteristics of currently tools used to probe receptor signaling. 23 Figure 2-1. The canonical receptor-GRK-arrestin cascade 26 Figure 2-2. BRET-based cAMP sensor CAMYEL 27 Figure 2-3. Comparison of forskolin and isoproterenol stimulation of cAMP in the CAMYEL assay. 28 Figure 2-4. PTX completely abolishes G protein activation. 29 Figure 2-5. PTX does not abolish arrestin recruitment. 30 Figure 2-6. GRK2 recruitment to D2R is negatively affected by PTX treatment. 30 Figure 2-7. Arrestin biased DREADD versus D2R. 33 Figure 2-8. G protein and arrestin recruitment to D2R wildtype and mutants. 35 Figure 2-10. The kinetics of arrestin recruitment to D2(L123W:R132L) relative to wildtype D2R. 36 Figure 2-9. D2(L123W:R132L) short and long isoforms behave similarly. 36 Figure 2-11. cAMPi by D2R wildtype and its mutants. 37 Figure 2-12. Arrestin mediated-internalization of D2R and its mutants. 38 Figure 2-13. Constitutive G protein activity for D2R and its mutants. 39 Figure 2-14. Schematic of AAV viral delivery system. 40 Figure 2-15. D2R(D114A) does not recruit G protein or arrestin. 40 Figure 2-16. Expression of D2R wildtype and its mutants in D2R-expressing MSNs in the NAc differentially affects basal locomotion and motivation tasks. 41 Figure 3-1. Comparison of modified S1 constructs. 46 Figure 3-2. S1(R9K:E129G) does not affect G protein signaling. 47 Figure 3-3. Schematic of AAV viral delivery system. 48 Figure 3-4. G protein biased D2R mutant (D2R GPB ). 49 Figure 3-5. D2-A4-mediated inhibition of cyclic AMP accumulation and G protein activation. 52 Figure 3-6. D2-A4-mediated arrestin3 recruitment. (A) Schematic of the BRET biosensor used to measure recruitment of arrestin to receptor. 53 Figure 3-7. D2-WT, D2-A3, and D2-A2-mediated G protein activation and arrestin3 recruitment. 55 ii Figure 3-8. Agonist-induced receptor internalization. 57 Figure 3-9. Characterization of quinpirole-induced recruitment of arrestin3. 58 Figure 3-10. Agonist-induced recruitment of GRK2. 59 Figure 3-11. Influence of GRK2 on receptor recruitment of arrestin3 and internalization. 61 Figure 3-12. Clathrin- and AP-2- mediated internalization of D2-WT. 62 Figure 3-13. Receptor mediated interaction between arrestin3 and β2-adaptin. 63 Figure 3-14. Interaction between receptors and β2-adaptin. 65 Table 3-1. pEC 50 values from G protein activation and recruitment assays 66 Figure 3-15. Arrestin recruitment to D2R requires the kinase activity of GRK2 or 6. 71 Figure 3-16. One hour preincubation of GRK inhibitors has no effect on arrestin recruitment. 72 Figure 3-17. Time course of arrestin recruitment to D2R and β2AR in the presence of a non-selective GRK inhibitor. 73 Figure 3-18. D2R IL3 truncation mutant is a supersignaling receptor. 75 Figure 3-19. D2R IL1 T->V mutant is impaired at G protein and arrestin signaling. 76 Figure 3-20. G protein activation and arrestin recruitment to IL mutants of D2R. 79 Figure 4-1. Aripiprazole differentially activates G protein-mediated pathways downstream of D2R. 82 Figure 4-3. Aripiprazole and 3508 do not activate GIRK channels downstream of D2R. 84 Figure 4-4. Theoretical unbiased GIRK activity of D2R ligands. 86 Figure 4-5. 3508 is not G protein versus arrestin biased. 88 Figure 4-6. Ligand-dependent conformational changes in Gi1 upon activation of D2R. 89 Figure 4-7. D2R activation by various D2R ligands promotes the interaction between Gβγ and GRKct. 90 Figure 4-8. D2R activation by various D2R ligands promotes the interaction between Gβγ and GIRK channels. 92 Figure 4-9. Optimization of the CODA-RET heterotrimeric G protein assay. 96 Figure 4-10. Subtype-specific G protein activation screen. 99 Figure 4-11. D2R-mediated GIRK activation is more highly correlated with Gαi1β5γ2 than Gαi1β1γ2 activation. 100 Figure 4-12. Characterization of the Gαi1β5γ2 heterotrimer. 102 Figure 4-13. Model of selective activation of cAMPi over GIRK by 3508 downstream of D2R. 104 Methods Figure 1. BRET-based arrestin assays. 133 iii Acknowledgements I first and foremost would like to thank my advisor, Dr. Jonathan Javitch, for years of unquantifiable mentorship, support and friendship. I would also like to thank Drs. Hideaki Yano and Eneko Urizar for their enthusiasm and guidance but, more importantly, their friendship. I would also like to thank the many current and past members of the Javitch, Kellendonk, Quick and Freyberg labs for the friendship and support. I am deeply indebted to Val Monrose for making sure the lab does not explode. I am very thankful for Douglas Drake, who is a constant source of thoughtfulness, laughter and delicious food. I would also like to thank Dr. Celine Gales, who was incredibly gracious for inviting me to train in her lab at INSERM in Toulouse, France. I would like to thank my collaborators, including Drs. Christoph Kellendonk, Zachary Freyberg, Kim Neve, Nevin Lambert, Vsevovold Gurevich, Lei Shi, Amy Newman, Robert Lane and Arthur Christopolous, as well as members of their labs. I would particularly like to thank Dr. Eduardo Gallo, who performed the behavioral analyses described below, Dr. Cecilea Clayton, who contributed significantly to the characterization of the D2-A3 mutant, and Dr. Mayako Michino for carrying modeling analyses on IL3 of D2R. I wish to thank my thesis committee (Drs. Jonathan Javitch, Christoph Kellendonk, Richard Mailman and Qin Fan) for their continual support throughout my graduate career. I would also like to thank my qualifying committee (Drs. Jonathan Javitch, Susan Steinberg, Qing Fan, and Lloyd Greene) as well as my mini-oral committee (Drs. Jonathan Javitch, Christoph Kellendonk and David Sulzer). I would also like to thank my thesis defense committee (Drs. Jonathan Javitch, Christoph Kellendonk, Richard Mailman, Nevin Lambert and Susan Steinberg) for their careful review of this manuscript. Many thanks to the Columbia University Pharmacology department (especially Drs. Robert Kass, Daniel Goldberg, Richard Robinson and Neil Harrison, and of course Karen Allis) for their support and creating positive academic environment. I would also like to thank the Pharmacology graduate students, especially Doug Barrows for his friendship and cat sitting support. iv Dedication I dedicate this thesis to many family and friends who have supported me throughout this process. This work is most of all dedicated to my wonderful and caring fiancé Erin Turner, who has been my inspiration. Her dedication to her work and to the people around her is awe-inspiring. I am forever in her debt for supporting me all of these years. And of course, I would like to dedicate this work to the queen of the household, our cat Pearl Turner-Donthamsetti. v CHAPTER 1. Introduction Schizophrenia is a neurodevelopmental disorder characterized by psychosis that manifests as hallucinations, delusions and/or disordered thought. 1 Features of this disorder also include negative symptoms (apathy, avolition, alogia) and cognitive deficits (deficits in working memory, processing speed, social cognition).
Details
-
File Typepdf
-
Upload Time-
-
Content LanguagesEnglish
-
Upload UserAnonymous/Not logged-in
-
File Pages160 Page
-
File Size-