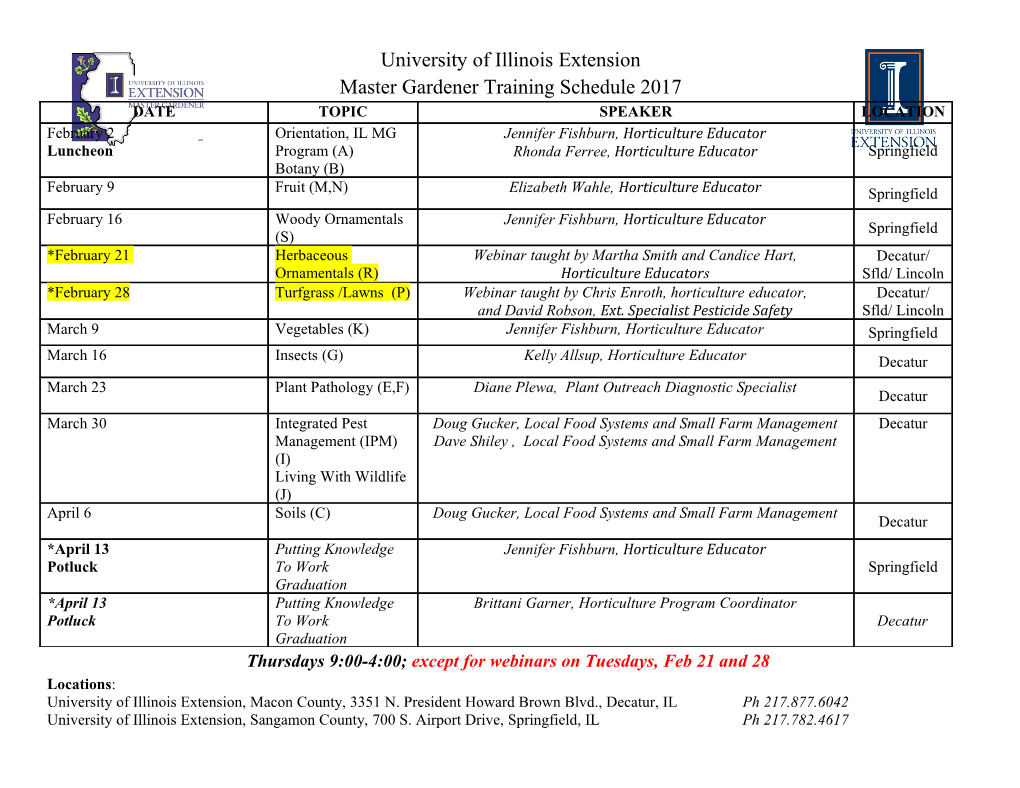
Evolutionary advantage of a broken symmetry in autocatalytic polymers tentatively explains fundamental properties of DNA Hemachander Subramanian1∗, Robert A. Gatenby1;2 1 Integrated Mathematical Oncology Department, 2Cancer Biology and Evolution Program, H. Lee Moffitt Cancer Center and Research Institute, Tampa, Florida. ∗To whom correspondence should be addressed; E-mail: hemachander.subramanian@moffitt.org. August 17, 2018 Abstract The macromolecules that encode and translate information in living systems, DNA and RNA, exhibit distinctive structural asymmetries, in- cluding homochirality or mirror image asymmetry and 30-50 directionality, that are invariant across all life forms. The evolutionary advantages of these broken symmetries remain unknown. Here we utilize a very simple model of hypothetical self-replicating polymers to show that asymmetric autocatalytic polymers are more successful in self-replication compared to their symmetric counterparts in the Darwinian competition for space and common substrates. This broken-symmetry property, called asym- metric cooperativity, arises with the maximization of a replication poten- tial, where the catalytic influence of inter-strand bonds on their left and right neighbors is unequal. Asymmetric cooperativity also leads to ten- tative, qualitative and simple evolution-based explanations for a number of other properties of DNA that include four nucleotide alphabet, three nucleotide codons, circular genomes, helicity, anti-parallel double-strand orientation, heteromolecular base-pairing, asymmetric base compositions, and palindromic instability, apart from the structural asymmetries men- tioned above. Our model results and tentative explanations are consistent with multiple lines of experimental evidence, which include evidence for the presence of asymmetric cooperativity in DNA. arXiv:1605.00748v3 [q-bio.BM] 9 Mar 2017 Introduction Living systems, uniquely in nature, acquire, store and use information au- tonomously. The molecular carriers of information, DNA and RNA, exhibit a number of distinctive physico-chemical properties that are optimal for in- formation storage and transfer[1]–[3]. This suggests that significant prebiotic evolutionary optimization[4] preceded and resulted in RNA and DNA, and that the nucleotide properties are not simply random. 1 Here, we concern ourselves with hypothetical self-replicating polymers that evolutionarily preceded DNA and RNA. Specifically, we imagine multiple species of autocatalytic polymers, constructed out of chemically-distinct monomers, competing for common precursors, energetic sources, catalytic surfaces and niches. Our central premise is that the simplest of the evolutionary strate- gies, higher rates of replication[5], determined the outcome of this evolutionary competition. We identify some fundamental, common-sense functional require- ments that autocatalytic polymers must satisfy in order to replicate faster than other competing species and hence be evolutionarily successful. The goal of this paper is to provide tentative and qualitative explanations for the structural and functional properties of DNA a posteriori as adaptations due to the evolutionary pressure to maximize replicational potential. Evidently, the evolutionary search for the perfect self-replicating molecular species in a given environment is constrained by the diversity of molecules avail- able to be used as monomers in that environment, in the primordial oceans. But, this constraint is intractable, in the absence of well-established knowledge of the chemistry of primordial oceans. We circumvent this biochemical constraint by ignoring its existence, and thus theoretically assume that evolution was allowed to experiment with an infinite variety of molecular species in its search for the perfectly-adapted monomer. This assumption translates into freedom for vari- ables and parameters describing the monomers to take on any value, in our mathematical model below. The above premise statement has its roots in the supervenience of evolution over chemistry. Its validation stems from its ability to tentatively explain multiple fundamental properties of DNA, as we will see below. The model In our simple phenomenological model of a primordial self-replicating system (Methods), we consider an autocatalytic polymer that is capable of replicating without the help of enzymes. A single strand of the polymer catalyzes the formation of another strand on top of itself, by functioning as the template. Free-floating monomers attach to the bound monomers on the template strand at lower temperatures, and facilitate covalent bonding between monomers[6] and hence polymerization, leading to the formation of the replica strand. The replica strand dissociates from the template strand at higher temperatures, creating two single strands, as happens in Polymerase Chain Reaction. A self-replicating molecular species must satisfy certain requirements in or- der to be evolutionarily successful and to function as an information-carrier. In the following, we list those physically meaningful requirements to be satisfied by the molecular species, and in doing so, arrive at two conflicting requirements. Breaking of a symmetry, upon maximization of replication potential, leads to resolution of the conflict and to simultaneous satisfaction of the two require- ments. These requirements are not new, and have been included and explored individually in other models and systems elsewhere[6]–[9]. Self-replication involves both bond formation between free-floating monomers and monomers on the template strand, and bond-breaking between monomers on the two strands, requiring these inter-strand bonds to be relatively weak com- pared to other bonds in the polymer. On the other hand, information storage 2 requires stronger intra-strand bonds that withstand strong environmental vari- ations, as pointed out by Schrödinger[10]. Hence, the self-replicating polymer needs to be composed of two complementary components, mutable inter-strand “hydrogen bonds” and relatively immutable intra-strand “covalent bonds”[6]–[9]. The intrinsic covalent bonding rates among free-floating monomers should be lower than the covalent bonding rates between the monomers hydrogen-bonded to the template strand, so that monomers become available for self-replication and not for de novo strand formation. This requirement makes self-replication viable and information transfer across generations possible. Evolution could have solved this by identifying monomers whose kinetic barrier for covalent bonding between themselves is lowered when they are attached to the template strand[6], [7], [11]. We term this barrier reduction “covalent bond catalysis”. If a hydrogen bond catalyzed the formation (and hence dissociation as well) of another hydrogen bond in its neighborhood[12], the strand would be replica- tionally more successful, since covalent bond formation requires two contiguous monomers hydrogen-bonded to the template. Also, higher rate of monomer attachment to the template would allow for more monomers to be drawn in for polymerization, away from other competing processes such as dimerization through hydrogen bonding. Thus, reduction of kinetic barrier for hydrogen bond formation would be advantageous for the self-replicating system. The foregoing justifies the need for “hydrogen bond cooperativity”, catalysis of hydrogen bond formation/dissociation by their neighboring hydrogen bonds[13]–[16]. Cooper- ativity in DNA, the increasing ease of hydrogen bonding between unbonded monomers (zippering) when two single DNA strands are already hydrogen- bonded at one of the ends, is a very well-established phenomenon, and has been well-studied both experimentally and theoretically[16]. The experimen- tal signature of cooperativity in DNA melting is the sharpness of the melting transition, where the DNA goes from a double strand to two single strands within a narrow range of temperature[17]. Cooperativity in DNA has also been abundantly documented in DNA zipping and unzipping experiments[18]–[21]. Obviously, the probability for the covalent bond formation between two con- tiguous monomers on the replica strand will increase with the lifetime of the hydrogen bonds of the monomers with the template strand. Thus, higher the kinetic barrier for hydrogen bond dissociation, higher the probability for the successful formation of the covalent bond and hence the replica strand. Thus, we notice that, while covalent bond catalysis requires higher kinetic barrier for hydrogen bond dissociation, hydrogen bond cooperativity requires lower kinetic barrier for hydrogen bond formation. Since self-replication requires the repli- cating polymer to be at or near the melting point of the hydrogen bonds, the kinetic barriers for formation and dissociation are nearly equal, and we arrive at the competing requirement of both higher and lower kinetic barrier height, or equivalently, to fine-tuning of the hydrogen bond lifetime. We could solve this conundrum by introducing an environment with oscillating ambient tempera- ture, where, the hydrogen bond lifetime is longer at lower temperatures and thus enables covalent bond formation, whereas, higher temperatures facilitate strand separation. Nevertheless, strands that intrinsically satisfy these two compet- ing requirements would still be evolutionarily more successful, by being able to colonize regions with temperature oscillations of much smaller amplitude. The solution that simultaneously and intrinsically satisfies these two com- peting
Details
-
File Typepdf
-
Upload Time-
-
Content LanguagesEnglish
-
Upload UserAnonymous/Not logged-in
-
File Pages41 Page
-
File Size-