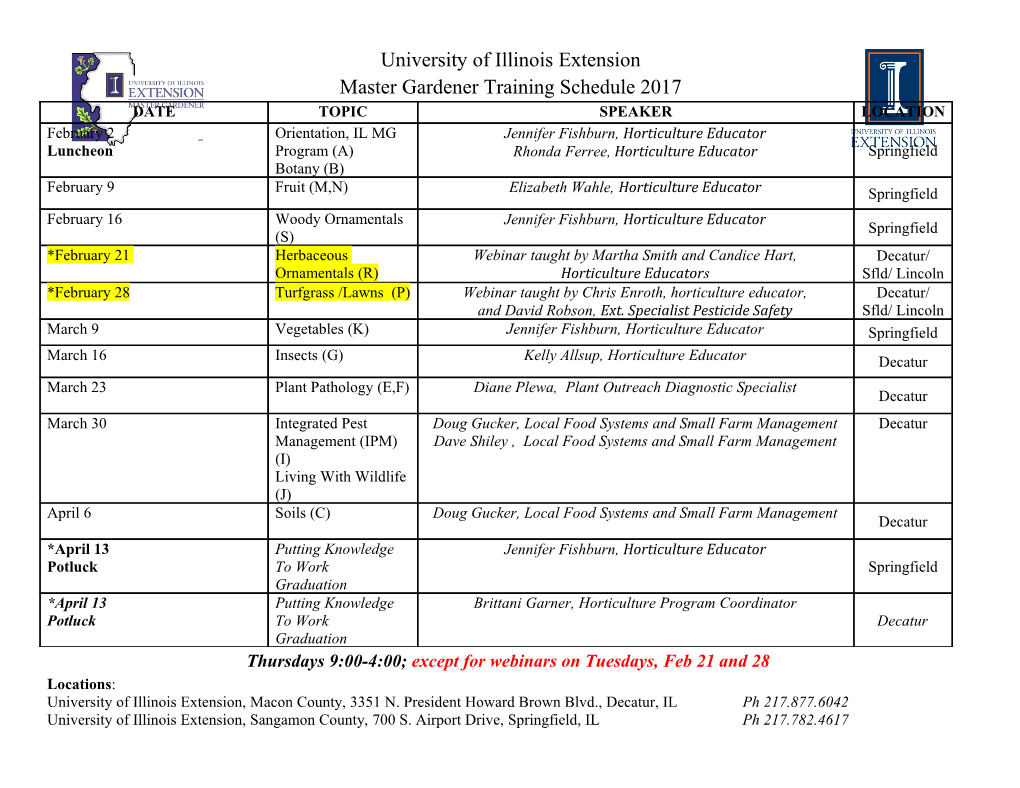
BARC/2011/E/003 BARC/2011/E/003 DEVELOPMENT OF A RAMAN SPECTROMETER TO STUDY SURFACE-ENHANCED RAMAN SCATTERING by Nandita Biswas, Ridhima Chadha, Sudhir Kapoor, Sisir K. Sarkar and Tulsi Mukherjee Radiation & Photochemistry Division 2011 BARC/2011/E/003 GOVERNMENT OF INDIA ATOMIC ENERGY COMMISSION BARC/2011/E/003 DEVELOPMENT OF A RAMAN SPECTROMETER TO STUDY SURFACE-ENHANCED RAMAN SCATTERING by Nandita Biswas, Ridhima Chadha, Sudhir Kapoor, Sisir K. Sarkar and Tulsi Mukherjee Radiation & Photochemistry Division BHABHA ATOMIC RESEARCH CENTRE MUMBAI, INDIA 2011 BARC/2011/E/003 BIBLIOGRAPHIC DESCRIPTION SHEET FOR TECHNICAL REPORT (as per IS : 9400 - 1980) 01 Security classification : Unclassified 02 Distribution : External 03 Report status : New 04 Series : BARC External 05 Report type : Technical Report 06 Report No. : BARC/2011/E/003 07 Part No. or Volume No. : 08 Contract No. : 10 Title and subtitle : Development of a Raman spectrometer to study surface-enhanced Raman scattering 11 Collation : 31 p., 15 figs. 13 Project No. : 20 Personal author(s) : Nandita Biswas; Ridhima Chadha; Sudhir Kapoor; Sisir K. Sarkar; Tulsi Mukherjee 21 Affiliation of author(s) : Radiation and Photochemistry Division , Bhabha Atomic Research Centre, Mumbai 22 Corporate author(s) : Bhabha Atomic Research Centre, Mumbai - 400 085 23 Originating unit : Radiation and Photochemistry Division, BARC, Mumbai 24 Sponsor(s) Name : Department of Atomic Energy Type : Government Contd... BARC/2011/E/003 30 Date of submission : January 2011 31 Publication/Issue date : February 2011 40 Publisher/Distributor : Head, Scientific Information Resource Division, Bhabha Atomic Research Centre, Mumbai 42 Form of distribution : Hard copy 50 Language of text : English 51 Language of summary : English, Hindi 52 No. of references : 36 refs. 53 Gives data on : 60 Abstract : Raman spectroscopy is an important tool, which provides enormous information on the vibrational and structural details of materials. This understanding is not only interesting due to its fundamental importance, but also of considerable importance in optoelectronics and device applications of these materials in nanotechnology. In this report, we begin with a brief introduction on the Raman effect and various Raman scattering techniques, followed by a detailed discussion on the development of an instrument with home-built collection optics attachment. This Raman system consists of a pulsed laser excitation source, a sample compartment, collection optics to collect the scattered light, a notch filter to reject the intense laser light, a monochromator to disperse the scattered light and a detector to detect the Raman signal. After calibrating the Raman spectrometer with standard solvents, we present our results on Surface- Enhanced Raman Scattering (SERS) investigations on three different kinds of chemical systems. 70 Keywords/Descriptors : RAMAN SPECTROSCOPY; RAMAN EFFECT; MONOCHROMATORS; SPECTROMETERS; ENERGY-LEVEL TRANSITIONS; PERFORMANCE TESTING 71 INIS Subject Category : S46 99 Supplementary elements : 1 Development of a Raman Spectrometer to Study Surface-Enhanced Raman Scattering Nandita Biswas, Ridhima Chadha, Sudhir Kapoor, Sisir K. Sarkar and Tulsi Mukherjee Radiation & Photochemistry Division Bhabha Atomic Research Centre, Mumbai 400085, India. Abstract Raman spectroscopy is an important tool, which provides enormous information on the vibrational and structural details of materials. This understanding is not only interesting due to its fundamental importance, but also of considerable importance in optoelectronics and device applications of these materials in nanotechnology. In this report, we begin with a brief introduction on the Raman effect and various Raman scattering techniques, followed by a detailed discussion on the development of an instrument with home-built collection optics attachment. This Raman system consists of a pulsed laser excitation source, a sample compartment, collection optics to collect the scattered light, a notch filter to reject the intense laser light, a monochromator to disperse the scattered light and a detector to detect the Raman signal. After calibrating the Raman spectrometer with standard solvents, we present our results on Surface-Enhanced Raman Scattering (SERS) investigations on three different kinds of chemical systems. 2 1. Introduction 1.1 The Raman Effect and Normal Raman Scattering The Raman effect arises when a photon is incident on a molecule and interacts with the electric dipole moment of the molecule [1]. In classical terms, the interaction can be viewed as a perturbation of the electric field of the molecule. When light i.e. the incoming photons interact with the electron cloud of the molecule, it results in most photons being elastically scattered (Rayleigh scattering). The incident and scattered photons, therefore, have the same energy (frequency) and wavelength. However, a small fraction of the scattered light (approximately 1 in 10 million photons) upon interaction with the sample is scattered at optical frequencies different from the frequency of the incident photons. During this scattering, the energy of the incoming photon is either red-shifted or blue-shifted, depending on the particular process. This phenomenon is called Raman scattering and was discovered by C.V. Raman [2] in 1928. Raman scattering provides a rich variety of information on the structure and composition of matter, based on its vibrational fingerprints. The vibrational information, which usually occurs at IR frequencies, can be obtained by monitoring the frequency shifts between the excitation and the scattered light. As a scattering process, however, the Raman effect is exceedingly weak: typical Raman cross sections per molecule range between 10-30 and 10-25 cm2 with the larger values occurring during resonant conditions, when the frequency of light happens to match an electronic transition in the molecule. By comparison, fluorescence spectroscopy, based on the absorption and emission of light, exploits effective cross sections between 10-17 and 10-16 cm2. However, the advent of the lasers as an intense and monochromatic source of excitation light was a milestone in the history of Raman spectroscopy and dramatically improved the strength of scattering signals. In quantum mechanics, the scattering phenomenon is described as an excitation to a virtual state lower in energy than a real electronic transition with instantaneous de-excitation to the final state [1]. The scattering event occurs in 10-14 second or less. The virtual-state description of scattering is shown in figure 1. When the energy of the incident photon (hvo) is the same as that of the scattered photon, the phenomenon is termed as Rayleigh scattering, as shown in figure 1(a). When a photon of energy hvo is absorbed by the molecule in the ground 3 vibrational state, part of the photon’s energy is transferred to the Raman active mode with frequency vm and the resulting frequency of the scattered light is reduced to vo-vm and is termed Stokes Raman scattering as shown in figure 1(b). At room temperature, the thermal population of vibrational excited states is low, although not zero. Therefore, for the majority of molecules, the initial state is the ground vibrational state, and the scattered photon will have lower energy (longer wavelength) than the exciting photon (Stokes shift). This Stokes shifted scatter is what is usually observed in Raman spectroscopy and is depicted in figure 1(b). According to the Boltzmann population of states, a small fraction of the molecules are in vibrationally excited states. Thus, when a photon of energy hvo is absorbed by a Raman-active molecule which at the time of interaction, is already in the excited vibrational state, excessive energy of the Raman active mode is released and the molecule returns to the ground vibrational state. The resulting frequency of scattered light goes up to vo+vm and is termed anti-Stokes Raman scattering as shown in figure 1(c). The anti-Stokes Raman scattering is always weaker than the Stokes scattering, but at room temperature it is strong enough to be useful for vibrational frequencies less than about 1500 cm-1. The Stokes and anti-Stokes spectra contain the same frequency information. The ratio of anti-Stokes to Stokes intensity at any vibrational frequency is a measure of temperature. anti-Stokes Raman scattering is useful for fluorescent molecules when the Stokes spectrum is masked by the fluorescence background. 1.2 Selection Rules and Intensities in Raman Spectroscopy The dipole moment, P, induced in a molecule by an external electric field, E, is expressed as P= E, where, is the polarizability of the molecule. The polarizability measures the ease with which the electron cloud around a molecule can be distorted. The induced dipole scatters light at the optical frequency of the incident light wave. The selection rule for a Raman-active vibration suggests that there will be a change in polarizability during the vibration, i.e. /Q ≠0, where, Q is the normal coordinate of the vibration. The scattering intensity is proportional to the square of the induced dipole moment, P2 i.e. (/Q)2. The observed strength of the Raman band is also proportional to the concentration of the species, as well as the intensity of the excitation laser. The main issue with Raman spectroscopy as mentioned earlier is the exceedingly weak scattering intensity. In order to get a detectable scattering intensity, it is necessary to enhance the 4 Raman scattering process
Details
-
File Typepdf
-
Upload Time-
-
Content LanguagesEnglish
-
Upload UserAnonymous/Not logged-in
-
File Pages31 Page
-
File Size-