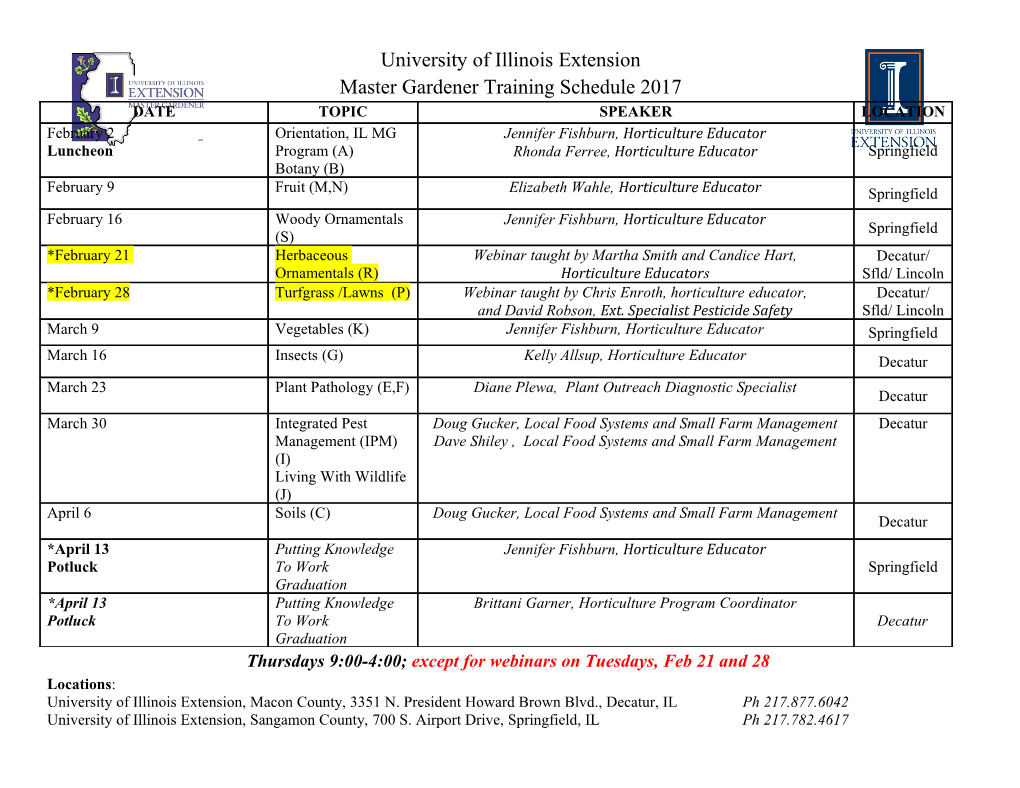
Supporting Information Chemical validation of Mycobacterium tuberculosis phosphopantetheine adenylyltransferase using fragment linking and CRISPR interference Jamal El Bakali1,†, Michal Blaszczyk2,†, Joanna C. Evans3, Jennifer A. Boland1, William J. McCarthy1, Marcio V. B. Dias2, Anthony G. Coyne1, Valerie Mizrahi3, Tom L. Blundell2, Chris Abell1,* and Christina Spry1,* 1Department of Chemistry, University of Cambridge, Lensfield Road, Cambridge CB2 1EW (UK) 2Department of Biochemistry, University of Cambridge, 80 Tennis Court Road, Cambridge CB2 1GA (UK) 3MRC/NHLS/UCT Molecular Mycobacteriology Research Unit, DST/NRF Centre of Excellence for Biomedical TB Research & Wellcome Centre for Infectious Diseases Research in Africa, Institute of Infectious Disease and Molecular Medicine and Department of Pathology, Faculty of Health Sciences, University of Cape Town, Anzio Road, Observatory 7925 (South Africa) †These authors contributed equally to this work *Correspondence: Chris Abell ([email protected]) or Christina Spry ([email protected]) Table of Contents Supplementary Figures……………………………………………………………………….2 Figure S1……………………………………………………………………………….2 Figure S2……………………………………………………………………………….3 Figure S3……………………………………………………………………………….4 Figure S4……………………………………………………………………………….5 Figure S5……………………………………………………………………………….6 Figure S6……………………………………………………………………………….7 Figure S7……………………………………………………………………………….8 Figure S8……………………………………………………………………………….9 Figure S9……………………………………………………………………………...10 Figure S10…………………………………………………………………………….11 Figure S11…………………………………………………………………………….12 Figure S12…………………………………………………………………………….13 Supplementary Schemes Scheme S1………………………………………………………………………….....14 Scheme S2…………………………………………………………………………….15 Scheme S3.……………………………………………………………………………16 Supplementary Table Table S1……………………………………………………………………………….17 Experimental Methods.………….…………………………………………………………..18 Expression and purification of MtbPPAT..……………………………………………18 Fluorescence-based thermal shift experiments………………………………………..19 Ligand-based NMR binding experiments……………………………………………..19 Isothermal titration calorimetry experiments…………………………………………20 X-ray crystallography…………………………………………………………………20 Mtb strains and growth conditions……………………………………………………21 Construction of CRISPRi conditional knockdown mutants…………………………..21 Effect of test compounds on Mtb growth……………………………………………..22 Synthetic organic chemistry…………………………………………………………..22 References……………………………………………………………………………………31 NMR spectra…………………………………………………………………………………32 1 Supplementary Figures ΔTm = +1.1 ± 0.2 °C ΔTm = +4.6 ± 0.5 °C ΔTm = +1.9 ± 0.2 °C ΔTm = +0.9 ± 0.1 °C ΔTm = +2.2 ± 0.5 °C ΔTm = +1.0 ± 0 °C ΔTm = +0.8 ± 0.2 °C ΔTm = +1.1 ± 0.1 °C ΔTm = +1.9 ± 0.2 °C ΔTm = +1.0 ± 0 °C ΔTm = +0.8 ± 0.2 °C ΔTm = +1.0 ± 0 °C ΔT = +1.0 ± 0.1 °C ΔT = +2.4 ± 0.1 °C ΔT = +1.0 ± 0 °C ΔT = +0.8 ± 0.1 °C m m m m Figure S1 : Structures and thermal shift values of the fragment hits identified from Figure S1: Structures and thermal shift (ΔTm) values (in ˚C) of the fragment hits identified in fluorescencea- basedfluorescence thermal-based thermal shift shiftscreening screen of (test1265 fragments concentration from a commercially : 5 mM) available library (Maybridge). All fragments were tested at a concentration of 5 mM. Fragments shown, reproducibly (in two independent experiments) increased the melting temperature of MtbPPAT by ≥ the 0.7 ˚C “hit” cut-off set. ΔTm values shown are averages from two independent experiments and errors represent range/2. 2 Active site binding confirmed by NMR Fragment hit No evidence of binding to MtbPPAT Figure S2: Analogues of fragment 1 tested for binding to MtbPPAT. Analogues were obtained from DuPont (6 – 8, S14 – S25) or purchased from commercial sources (4, 5 and S26 – S28). Active site binding was confirmed using WaterLOGSY and STD NMR, with ligands present at 1 mM and MtbPPAT at 20 µM; active site displacement was achieved using 1 mM ATP or CoA. *Fragments S26 – S28 were tested for active site binding by WaterLOGSY and STD NMR; other fragments without evidence of binding to MtbPPAT were tested (at a concentration of 5 mM) by thermal shift assay only. 3 No evidence Active site binding confirmed by NMR of binding to MtbPPAT Fragment hit Figure S3: Analogues of 2 tested for binding to MtbPPAT. Analogues 9-11 were synthesised according to Scheme S2, while analogues 12, 13 and S29 – 31 were purchased from commercial sources. Active site binding to MtbPPAT was probed using ligand-based NMR experiments (WaterLOGSY and STD), with ligands present at 1 mM and MtbPPAT at 20 µM; active site displacement was achieved using 1 mM ATP or CoA. 4 Active site binding confirmed by NMR Fragment hit No evidence of binding to MtbPPAT Figure S4: Analogues of fragment 3 tested for binding to MtbPPAT. Analogues 14, S52 and S53 were synthesised according to Scheme S3, while analogues 17 and 18 were purchased from commercial sources and analogues 15 – 16, S31 – S51 and S54 were obtained from DuPont. Active site binding was confirmed using ligand-based NMR experiments (WaterLOGSY and STD), with ligands present at 1 mM and MtbPPAT at 20 µM; active site displacement was achieved using 1 mM ATP or CoA. *Fragments S52 and S53 were tested for active site binding by ligand-based NMR experiments (WaterLOGSY and STD); other fragments without evidence of binding to MtbPPAT were tested (at a concentration of 5 mM) by thermal shift assay only. 5 9 July 2020 version A) Time (min) B) Time (min) C) Time (min) /sec cal µ CoA to Mtb PPAT CoA to Mtb PPAT + CoA to Mtb PPAT + 10 mM compound 2 10 mM compound 3 KD app(CoA) = 35 µM KD(CoA) = 2.9 µM KD(2) = 0.9 mM kcal/mole of injectant LE(2) = 0.28 Molar Ratio Molar Ratio Molar Ratio Figure S5: ITC analysis of MtbPPAT binding to CoA, indole fragment 2 and pyrazole fragment 3. Titration of MtbPPAT with 600 µM CoA in the absence (A) or presence of 10 mM indole fragment 2 (B) or pyrazole fragment 3 (C). In A and B, the initial concentration of MtbPPAT was 54 µM and in C, the initial concentration was 72 µM (protomer concentrations, equivalentC) to 9 and 12 µM hexamer, respectivelyD) ). The upper panels show the change in energy required to maintain a constant temperature during the titration and the bottom panels show the integrated heats of binding. Titrations were performed in the presence of 30 mM HEPES, pH 8.0, 200 mM NaCl, 5 mM MgCl2, 0.5 mM TCEP and 5% (v/v) DMSO. To the solution of MtbPPAT, 19 ´ 2 µL test compound was injected. In C, where saturation of the protein was 19 injections not achieved after 19 injections, a further 19 ´ 2 µL test compound was injected. Data in A and B are fitted to a “one set of sites” binding model (solid line). In A and B, binding stoichiometries (n) of 0.7 (relative to the protomer) were determined. The apparent KD (KD app) removed determined for CoA in the presence of fragment 2 was used to calculate a KD for fragment 2 (as described in the experimental methods). The corresponding LE of indole fragment 2, in kcal.mol-1.NHA-1, is shown. KD app(CoA) = 32 µM KD(Cpd 12) = 1.0 mM LE(Cpd 12) = 0.28 6 A) * Reporter 3 - Mtb PPAT B) 3 + Mtb PPAT * 3 C) 3 + Mtb PPAT + 15 D) 3 + Mtb PPAT + 16 E) 3 + Mtb PPAT + 18 F) Increasing 3 + Mtb PPAT + 17 affinity of competitor 3 + 2 ppmMtb PPAT + 14 1 δ H Figure S6: Ranking of pyrazole fragments based on affinity by competition-based STD-NMR. STD-NMR spectra showing the STD signal intensity of the C5-methyl protons (*) of pyrazole 3 (1 mM; the “reporter” molecule) in the absence (A) or presence (B-F) of 20 µM MtbPPAT (protomer concentration, equivalent to 3.3 µM hexamer) and absence (A-B) or presence of 1 mM “competitor” fragments: pyrazole 15 (C), 16 (D), 18 (E) and 17 (F). The rank order of affinity inferred for the competitor molecules based on the STD signal of the reporter molecule, is shown. 7 10 July 2020 version - Figure S6 Time (min) Time (min) /sec cal µ CoA to Mtb PPAT CoA to Mtb PPAT + 10 mM compound 12 KD app(CoA) = 32 µM KD(CoA) = 2.9 µM KD(12) = 1.0 mM kcal/mole of injectant LE(12) = 0.29 Molar Ratio Molar Ratio Figure S7: Competitive ITC analysis of MtbPPAT binding to indole fragment 12. Titration of MtbPPAT with 600 µM CoA in the absence (LHS) or presence of 10 mM indole fragment 12 (RHS). The initial concentration of MtbPPAT was 54 µM (protomer concentration, equivalent to 9 µM hexamer). The upper panels show the change in energy required to maintain a constant temperature during the titration and the bottom panels show the integrated heats of binding. Titrations were performed in the presence of 30 mM HEPES, pH 8.0, 200 mM NaCl, 5 mM MgCl2, 0.5 mM TCEP and 5% (v/v) DMSO. To the solution of MtbPPAT, 19 ´ 2 µL test compound was injected. Data are fitted to a “One Set of Sites” binding model (solid line). Binding stoichiometries (n) of 0.5 – 0.7 were determined. The LE of indole fragment 12, in kcal.mol-1.NHA-1, is shown. 8 Version revised on 9/8/20 H O A N HB HO N HC O HB HD HF HC HG HE 20 HH N H HI A) 20 – MtbPPAT B) HB-D, F 20 + MtbPPAT HA HI HH HG HE C) 20 + MtbPPAT + CoA 8.0 7.5 7.0 ppm 1 δ H Figure S8: Detection of CoA-competitive binding of compound 20 to MtbPPAT by STD- NMR. STD-NMR spectra showing the STD signals (or lack thereof) of the aromatic protons of compound 20 in the absence (A) or presence (B-C) of 20 µM MtbPPAT (protomer concentration, equivalent to 3.3 µM hexamer) and absence (A-B) or presence of 1 mM CoA (C). 9 5 August 2020 version - Figure S9 Time (min) 0 25 50 75 100 O 0 HO -0.04 /sec -0.08 cal µ O N -0.12 N -0.16 19 to MtbPPAT 0 N H -0.48 19 -0.96 KD = 38 ± 2 µM LE = 0.23 kcal/mole of injectant 0 1 2 3 Molar Ratio Figure S9: ITC analysis of MtbPPAT binding to compound 19.
Details
-
File Typepdf
-
Upload Time-
-
Content LanguagesEnglish
-
Upload UserAnonymous/Not logged-in
-
File Pages42 Page
-
File Size-