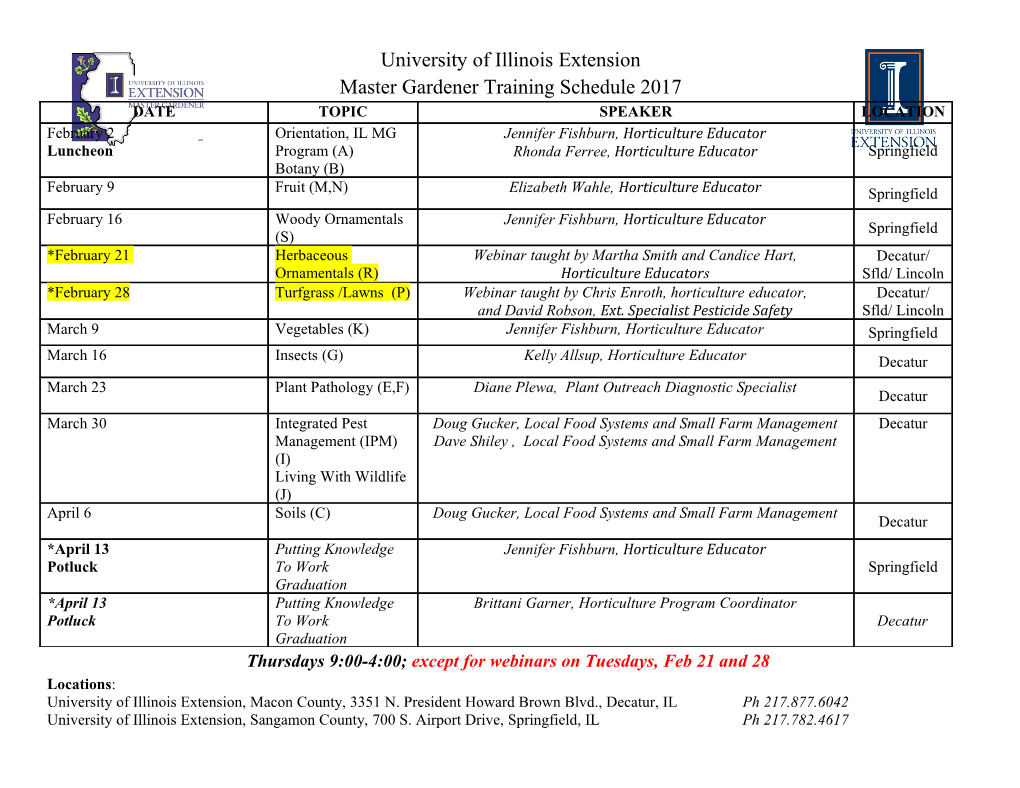
SHOCKWAVE ENERGY DISSIPATION BY MECHANORESPONSIVE MATERIALS BY JAEJUN LEE DISSERTATION Submitted in partial fulfillment of the requirements for the degree of Doctor of Philosophy in Materials Science and Engineering in the Graduate College of the University of Illinois at Urbana-Champaign, 2018 Urbana, Illinois Doctoral Committee: Professor Nancy R. Sottos, Chair and Director of Research Professor Kenneth S. Schweizer Assistant Professor Cecilia Leal Assistant Professor Christopher M. Evans ABSTRACT Shockwaves are characterized by high pressure and strain rate with extremely short duration about nanoseconds. Blast-induced traumatic brain injury sustained by personnel exposed to shockwave-generating high-energy explosives results in costly and debilitating health effects. Novel approaches for shockwave energy dissipation (SWED) are demanded since conventional impact absorbing materials do not provide sufficient shockwave attenuation. This research seeks to develop new approaches for efficient SWED in materials designed based on the availability of shock responsive chemical and physical reactions. A laser-induced shockwave test is developed to evaluate the energy dissipation performance of testing films. For a glass substrate, the input pressure of the shockwave with a Gaussian shaped pressure profile is tuned from 1 to 2 GPa by adjusting launch laser fluence. A fused silica substrate develops an initial stress wave to a triangle shaped pressure profile due to the nonlinear wave propagation properties, which allows the exploration of the duration time effects on energy dissipation. Benchmark energy dissipation values are obtained from polyurea films to provide a standard of comparison. The availability of energy dissipation by shock-induced microstructural changes and chemical reactions is investigated. In a series of network-forming ionic liquids (NILs) synthesized, each NIL possesses a different nano-scale structure consisting of charged clusters and alkyl domains. Irreversible shock-induced structural ordering changes with the creation of new nano-segregated domains in the NIL with the longest alkyl chain spacer enhances the shockwave absorption performance. The more ordered domains result in an 11.2 K increase of Tg due to the extra spatial restriction. In another set of experiments, the energy dissipation properties of the dynamic PDMS networks (PDMS-B-DR) containing boronic ester bonds, which allows self-healing via reversible ii bond exchange reactions are studied. By increasing the density of boronic ester bonds in PDMS- B-DR, lower peak pressures are measured, and its energy absorption capability outperforms covalent PDMS and polyurea. PDMS7-B-DR with the highest density of boronic ester bonds reduces the input peak pressure to 19.9 % at the highest launch laser fluence. The dissociation of boronic ester bonds in PDMS-B-DR is assumed as the main mechanism for energy dissipation. Finally, the potential of using a laser-induced shockwave to facilitate the phase transformation of supercooled liquids is explored. The accelerated nucleation of 1,2-bis(phenylethynyl)benzene (PEB) from its supercooled state is induced by shockwave impact with 1.2 GPa peak pressure and 15 ns duration. The nano-scale structural effects on shockwave energy dissipation is explored using a series of polymerized ionic liquids (PILs) with varying alkyl spacer length between imidazolium cations. X-ray scattering analysis reveals that each of the amorphous PILs exhibit distinct nanoscale structural heterogeneity, depending on the length of the chain spacer. Although the morphology is different, the PILs are designed and synthesized to have similar glass transition temperature. Increased structural heterogeneity in the PILs, corresponding to more ordered charged clusters, leads to greater energy dissipation. In addition, we observe the amorphous phase is more effective at attenuating energy than the crystalline phase due to close packed morphology and slow kinetics. The shock-induced chemical reactions and nano-scale structural changes observed from the mechanoresponsive materials in this dissertation provide new insights for the development of efficient shockwave absorption materials that prevent traumatic brain injuries. The nano-scale heterogeneous structure consisting of two different phases is critical for designing soft materials for effective dissipation of shockwave loading. iii ACKNOWLEDGMENTS First, I want to express my sincere appreciation to my research advisor, Professor Nancy Sottos, who has guided me to finish my dissertation study throughout the last six years. Her passion on the fields of science and engineering has influenced me to pursue a career in academic research and driven me to become a real scientist. I would also like to thank my thesis committee, specifically Professors Kenneth Schweizer, Cecilia Leal, and Christopher Evans for their advices and discussions, which have allowed me to succeed in graduate studies. I would like to thank all of AMS and Sottos/White research group colleagues for the helps and discussions. They taught me how sharing ideas is valuable for the successful research. My collaborators, Ke Yang, Yi Ren, and Vivian Lau, in the field of chemistry provided me materials and presented knowledge to drive my research forward. I could not have finished the dissertation study without love from my parents. They always encourage me to embrace challenges and support my life decisions. I also want to thank all my friends in Champaign/Urbana who made my life healthy and fruitful during the six years. I would like to acknowledge the Office of Naval Research for the funding of my graduate studies. iv TABLE OF CONTENTS CHAPTER 1 INTRODUCTION ...................................................................................................1 1.1 Blast-induced Traumatic Brain Injuries ........................................................................... 1 1.2 Shockwave Dissipation Mechanisms ............................................................................... 3 1.2.1 Impedance mismatch technique ................................................................................ 3 1.2.2 Shockwave-induced chemical reaction ..................................................................... 6 1.2.3 Shockwave-induced physical reaction ...................................................................... 6 1.3 Polyurea for Shockwave Mitigation ................................................................................. 8 1.4 Measurement Techniques for Shockwave Energy Dissipation ...................................... 12 1.5 Overview of Thesis Research ......................................................................................... 14 1.6 References ...................................................................................................................... 16 CHAPTER 2 LASER-INUDCED SHOCKWAVE TEST ..........................................................21 2.1 Introduction .................................................................................................................... 21 2.2 Experimental Methods ................................................................................................... 22 2.2.1 Laser-induced shockwave test setup and protocols ................................................ 22 2.2.2 Input shockwave pressure measurement ................................................................. 25 2.2.3 Preparation of polyurea specimen ........................................................................... 26 2.3 Results and Discussions ................................................................................................. 26 2.3.1 Tunable input shockwave pressure and duration time ............................................ 26 2.3.2 Shockwave energy dissipation by polyurea ............................................................ 29 2.4 Conclusions .................................................................................................................... 30 2.5 References ...................................................................................................................... 31 CHAPTER 3 SHOCK-INDUCED ORDERING OF NETWORK-FORMING IONIC LIQUIDS ..33 3.1 Introduction .................................................................................................................... 33 3.2 Experimental Methods ................................................................................................... 34 3.2.1 Materials and synthesis ........................................................................................... 34 3.2.2 Sample preparation for laser-induced shockwave test ............................................ 35 3.2.3 Protocol for repeated shockwave testing ................................................................ 36 3.2.4 Characterization of NILs......................................................................................... 36 3.3 Results and Discussions ................................................................................................. 37 3.3.1 Shockwave energy dissipation by NILs .................................................................. 37 v 3.3.2 Shock-induced ordering in NILs ............................................................................. 39 3.4 Conclusions .................................................................................................................... 45 3.5 References .....................................................................................................................
Details
-
File Typepdf
-
Upload Time-
-
Content LanguagesEnglish
-
Upload UserAnonymous/Not logged-in
-
File Pages110 Page
-
File Size-