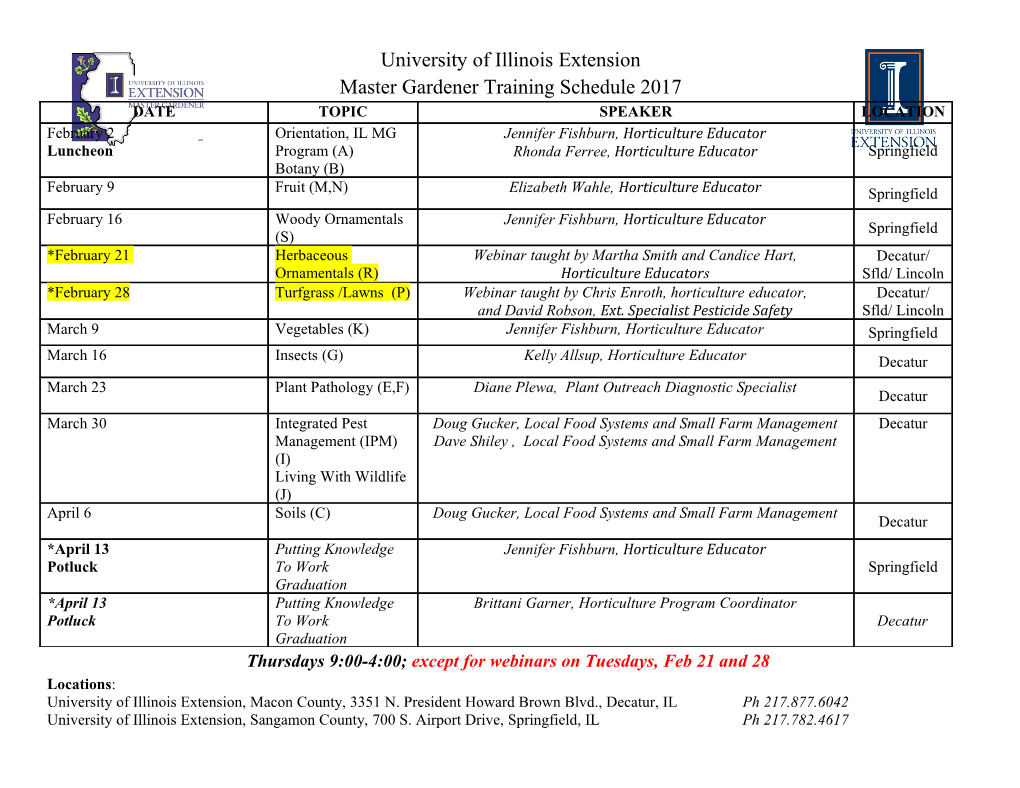
3 The Laplace Equation Introduction – Well Posed Problems. Uniqueness – Harmonic Functions – Fundamental Solution and Newtonian Potential – The Green Function – Uniqueness in Unbounded Domains – Surface Potentials 3.1 Introduction The Laplace equation Δu = 0 occurs frequently in applied sciences, in particular in the study of the steady state phenomena. Its solutions are called harmonic functions. For instance, the equilibrium position of a perfectly elastic membrane is a harmonic function as it is the velocity potential of a homogeneous fluid. Also, the steady state temperature of a homogeneous and isotropic body is a harmonic function and in this case Laplace equation constitutes the stationary counterpart (time independent) of the diffusion equation. Slightly more generally, Poisson’s equation Δu = f plays an important role in the theory of conservative fields (electrical, magnetic, gravitational,...) where the vector field is derived from the gradient of a potential. For example, let E be a force field due to a distribution of electric charges in a domain Ω ⊂ R3. Then, in standard units, div E =4πρ,whereρ represents the density of the charge distribution. When a potential u exists such that ∇u = −E, then Δu =div∇u = −4πρ, which is Poisson’s equation. If the electric field is created by charges located outside Ω,thenρ =0inΩ and u is harmonic therein. Analogously, the potential of a gravitational field due to a mass distribution is a harmonic function in a region free from mass. In dimension two, the theories of harmonic and holomorphic functions are strictly connected1. Indeed, the real and the imaginary part of a holomorphic 1 A complex function f = f (z)isholomorphic in an open subset Ω of the complex plane if for every z0 ∈ Ω, the limit f (z) − f (z0) lim = f (z0) z→z0 z − z0 Salsa S. Partial Differential Equations in Action: From Modelling to Theory c Springer-Verlag 2008, Milan 3.2 Well Posed Problems. Uniqueness 103 function are harmonic. For instance, since the functions zm = rm (cos mθ + i sin mθ) ,m∈ N, (r, θ polar coordinates) are holomorphic in the whole plane C, the functions u (r, θ)=rm cos mθ and v (r, θ)=rm sin mθ m ∈ N, are harmonic in R2 (called elementary harmonics). In Cartesian coordinates, they are harmonic polynomials; for m =1, 2, 3 we find x, y, xy, x2 − y2,x3 − 3xy2, 3x2y − y3. Other examples are u (x, y)=eαx cos αy, v (x, y)=eαx sin αy (α ∈ R), the real and imaginary parts of f (z)=eiαz, both harmonic in R2,and u (r, θ)=logr, v (r, θ)=θ, ≡ R2\ the real and imaginary parts of f (z)=log0 z log r + iθ,harmonicin (0, 0) and R2\{θ =0} , respectively. In this chapter we present the formulation of the most important well posed problems and the classical properties of harmonic functions, focusing mainly on dimensions two and three. As in Chapter 2, we emphasize some probabilistic as- pects, exploiting the connection among random walks, Brownian motion and the Laplace operator. A central notion is the concept of fundamental solution, that we develop in conjunction with the very basic elements of the so called potential theory. 3.2 Well Posed Problems. Uniqueness Consider the Poisson equation Δu = f in Ω (3.1) where Ω ⊂ Rn is a bounded domain. The well posed problems associated with equation (3.1) are the stationary counterparts of the corresponding problems for the diffusion equation. Clearly here there is no initial condition. On the boundary ∂Ω we may assign: • Dirichlet data u = g, (3.2) exists and it is finite. 104 3 The Laplace Equation • Neumann data ∂ν u = h, (3.3) where ν is the outward normal unit vector to ∂Ω, • a Robin (radiation) condition ∂ν u + αu = h (α>0), (3.4) • a mixed condition; for instance, u = g on ΓD (3.5) ∂ν u = h on ΓN , where ΓD ∪ ΓN = ∂Ω, ΓD ∩ ΓN = ∅,andΓN is a relatively open subset of ∂Ω. When g = h = 0 we say that the above boundary conditions are homogeneous. We give some interpretations. If u is the position of a perfectly flexible mem- brane and f is an external distributed load (vertical force per unit surface), then (3.1) models a steady state. The Dirichlet condition corresponds to fixing the position of the membrane at its boundary. Robin condition describes an elastic attachment at the boundary while a homogeneous Neumann condition corresponds to a free vertical motion of the boundary. If u is the steady state concentration of a substance, the Dirichlet condition prescribes the level of u at the boundary, while the Neumann condition assigns the flux of u through the boundary. Using Green’s identity (1.13) we can prove the following uniqueness result. Theorem 3.1. Let Ω ⊂ Rn be a smooth, bounded domain. Then there exists at most one solution u ∈ C2 (Ω) ∩ C1 Ω of (3.1), satisfying on ∂Ω one of the conditions (3.2), (3.4) or (3.5). In the case of the Neumann condition, that is when ∂ν u = h on ∂Ω, two solutions differ by a constant. Proof. Let u and v be solutions of the same problem, sharing the same boundary data, and let w = u − v.Thenw is harmonic and satisfies homogeneous boundary conditions (one among (3.2)-(3.5)). Substituting u = v = w into (1.13) we find 2 |∇w| dx = w∂ν wdσ. Ω ∂Ω If Dirichlet or mixed conditions hold, we have w∂νwdσ=0. ∂Ω 3.3 Harmonic Functions 105 When a Robin condition holds 2 w∂νwdσ= − αw dσ ≤ 0. ∂Ω ∂Ω In any case we obtain that |∇w|2 dx ≤0. (3.6) Ω From (3.6) we infer ∇w = 0 and therefore w = u − v = constant. This concludes the proof in the case of Neumann condition. In the other cases, the constant must be zero (why?), hence u = v. Remark 3.1. Consider the Neumann problem Δu = f in Ω, ∂ν u = h on ∂Ω. Integrating the equation on Ω and using Gauss’ formula we find fdx = hdσ. (3.7) Ω ∂Ω The relation (3.7) appears as a compatibility condition on the data f and h,that has necessarily to be satisfied in order for the Neumann problem to admit a so- lution. Thus, when having to solve a Neumann problem, the first thing to do is to check the validity of (3.7). If it does not hold, the problem does not have any solution. We will examine later the physical meaning of (3.7). 3.3 Harmonic Functions 3.3.1 Discrete harmonic functions In Chapter 2 we have examined the connection between Brownian motion and diffusion equation. We go back now to the multidimensional symmetric random walk considered in Section 2.6, analyzing its relation with the Laplace operator Δ. For simplicity we will work in dimension n = 2 but both arguments and conclusions may be easily extended to any dimension n>2. We fix a time step τ>0, a space 2 step h>0 and denote by hZ the lattice of points x =(x1,x2) whose coordinates are integer multiples of h.Letp (x,t)=p (x1,x2,t) be the transition probability function, giving the probability to find our random particle at x at time t.From the total probability formula we found a difference equation for p,thatwerewrite in dimension two: 1 p (x,t+ τ)= {p (x+he ,t)+p (x−he ,t)+p (x+he ,t)+p (x−he ,t)} . 4 1 1 2 2 (3.8) We can write this formula in a more significant way by introducing the mean value operator Mh, whose action on a function u = u(x) is defined by the following 106 3 The Laplace Equation formula: 1 M f (x)= {u (x+he )+u (x−he )+u (x+he )+u (x−he )} h 4 1 1 2 2 1 = u (y) . 4 |x−y|=h 2 Note that Mhu (x) gives the average of u over the points of the lattice hZ at distance h from x. We say that these points constitute the discrete neighborhood of x of radius h. It is clear that (3.8) can be written in the form p (x,t+ τ)=Mhp (x,t) . (3.9) In (3.9) the probability p at time t + τ is determined by the action of Mh at the previous time, and then it is natural to interpret the mean value operator as the generator of the random walk. Now we come to the Laplacian. If u is twice continuously differentiable, it is not difficult to show that2 M u (x) − u (x) 1 lim h → Δu (x) . (3.10) h→0 h2 4 The formula (3.10) induces to define, for any fixed h>0, a discrete Laplace operator through the formula ∗ − Δh = Mh I ∗ where I denotes the identity operator (i.e. Iu = u). The operator Δh acts on functions u defined in the whole lattice hZ2 and, coherently, we say that u is ∗ d-harmonic (d for discrete)ifΔhu =0. Thus, the value of a d-harmonic function at any point x is given by the average of the values at the points in the discrete neighborhood of x of radius h. We can proceed further and define a discrete Dirichlet problem. Let A be a subset of hZ2. We say that A is connected if, given any couple of points x0, x1 in A,itis possible to connect them by a walk3 on hZ2 entirely contained in A. Moreover, we say that x ∈A is an interior point of A if its h−neighborhood is contained in A.ThepointsofA that are not interior are called boundary points (Fig.
Details
-
File Typepdf
-
Upload Time-
-
Content LanguagesEnglish
-
Upload UserAnonymous/Not logged-in
-
File Pages54 Page
-
File Size-