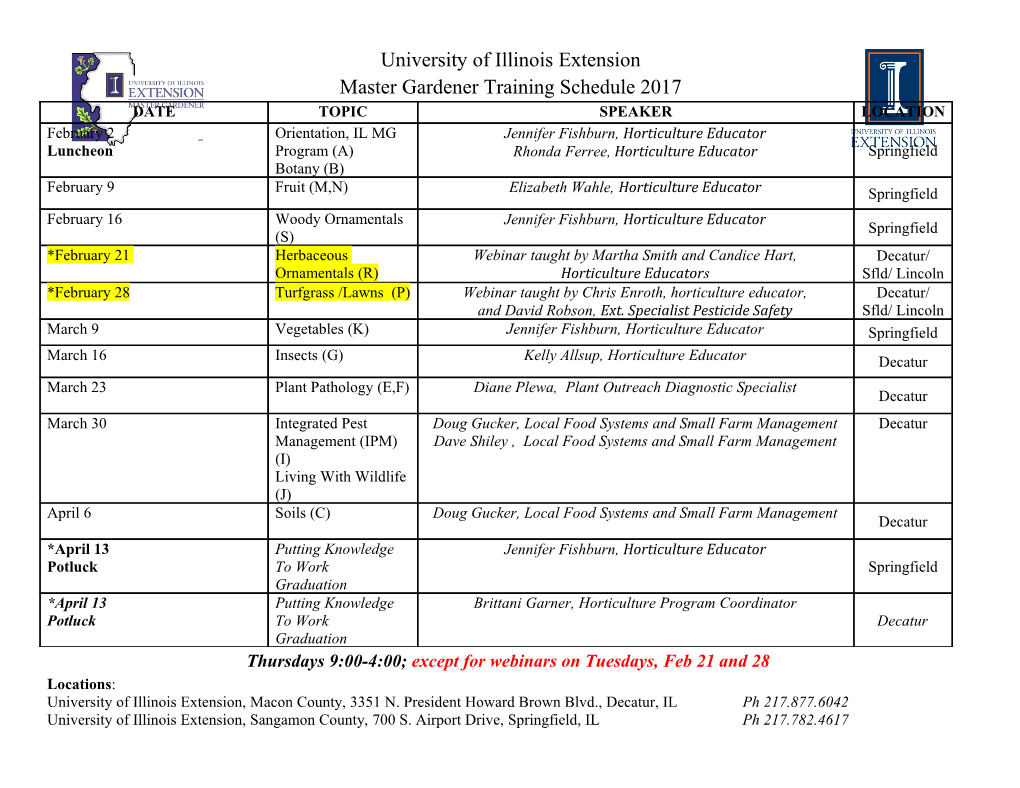
Journal of the Geological society, London, Vol. 144, 1987, pp. 281-297, 13 figs. Printed in Northern Ireland The segregation and emplacement of granitic magmas S. M. WICKHAM Division of Geological and Planetary Sciences California Institute of Technology, Pasadena, California 91125 USA Abstract: The segregation of granitic magma from residual crystals at low melt-fraction is strongly dependent on the viscosity of the melt. Theoretical considerations imply that for the typical range of granitic meltviscosities (104Pa S to 10” Pa S) only verylimited separation will bepossible by a compaction mechanism over the typical duration of a crustal melting event (c. 106years). Small-scale segregations (millimetre to metre) of the type observed in migmatite terranes may be generated by compaction (possiblyassisted by -continuousdeformation), or byflow of melt into extensional fractures, but low melt-fraction liquids are unlikely to be extracted to form large (kilometre-size) granitic plutons because of the limited separation efficiency. At higher melt-fractions (>30%) the rapid decrease in strengthand effectiveviscosity during partial meltingallows other segregation processes tooperate. Calculations and experiments indicate that in granitic systems the effective viscosity of partially melted rocks,having a very narrow melt fraction range of 30-50% will fall rapidly to levels at which convective overturn of kilometre-thick zones can occur. Convective motion within anatectic regionsis capable of generating large (kilometre-size) homogeneous, highcrystal- fraction, crustally-derived magma bodies, which are orders of magnitude greater insize than low melt-fraction segregates. Before convective instability is reached, small (centimetre- to metre-sized) pods of granitic liquid may rise buoyantly through, and pond at the top of such partly molten zones; such a process is consistent with the observation that some granulites appear to be residue rocks, chemically depleted in a minimum melt component. The effective viscosity (and hence the suscep- tibility to convection) of a partially melted zone within the crust, is strongly dependent on the water content of the system at a given pressure and temperature, because this controls both the quantity of melt generatedand also the viscosity of the melt. The intrinsic water content of mostcrustal lithologies is incapable of promoting the high percentages of partial melting, or the lowliquid viscosities, required to form large kilometre-sized granitic plutons by convective homogenization, at typical crustal temperatures. This suggests thatthe anatexis involvedin the generation of large crustally-derived magma bodies has in many cases been promoted by an influx of externally derived aqueous fluid. These magma segregation processes are illustrated with respect to the petrogenesis of three different types of granitoid pluton from a Hercynian low-pressure, metamorphic-anatectic terrane in the Pyrenees Crustal melting is fundamental to the generation of most used to generate silicic melts from crustal rocks, (e.g. Hoffer granitic plutons (e.g. Tuttle & Bowen 1958; Wyllie 1977), 1978; Winkler 1979; Wickham 1984; Johannes 1985) and in yet there is very little detailed understanding of the many some cases have helped to confirm an anatectic origin for processes involved between the inception of anatexis and graniticsegregations. The heterogeneouscharacter of the emplacement of plutons at high levels in the crust. While migmatites is typically on a scale of 1 cm to 1 m although recognizing that contamination of mantle-derived mafic these are notrigorous limits. Granitic melt segregations magmas with crustalmaterial may be animportant within migmatite terranes are therefore ona small scale, five granite-forming process, this paper will be concerned with or six orders of magnitudesmaller in size than typical the origin of those granites primarily derived by crustal granitic plutons. anatexis (e.g. Allegre & Ben Othman 1980; Hamilton et al. Studies of granitic magma bodies have used petrological, 1980; Vitrac-Michard et al. 1980; Farmer & DePaolo 1983; geochemical and fluid dynamic approaches (e.g. Shaw 1965; Chappell 1984; Frost & O’Nions 1985). There havebeen Hildreth 1981; White & Chappell 1977; Huppert et al. 1982; two main approaches to the study of such melting processes. Marsh 1982; Chappell 1984; Chappell et al. inpress). In One of these is the study of the low melt-fraction rocks contrast to migmatite research, these studies have dealt with (<40% melt) represented by migmatite terranes.The high melt-fraction rocks where physical properties are second involves the study of silicic magmas (i.e. high controlled dominantly by the properties of the liquid part of melt-fractionrocks containing >40% melt) and their the system, in particular the liquid viscosity. Viscosity in differentiation, emplacement and eruption histories. granitic liquids at normal crustal temperatures depends most In petrologicalstudies of migmatites (reviewed in importantly on water content,temperature and bulk Ashworth 1985), mineral phase equilibria can often be used composition but also on pressure (which strongly influences to constrain the physico-chemical conditions within specific water solubility). (In this paper, magma refers to silicate terranes (P, T, aHZ0), (e.g. Tyler & Ashworth 1982; melt containingsuspended crystals; liquid refers to McLellan 1984). Major and trace element geochemical data crystal-free silicate melt.) However, viscosity is complicated can alsoconstrain melt-fractions (e.g. Barr 1985) and by the fact that most bodies of granitic magma contain identify migmatite source rocks (e.g. Brown 1979; Yardley suspended crystals for part or all of their history. In this et al. 1987; Wickham 1987). Melting experiments have been paper particular attention is given to the dramatic change in 281 Downloaded from http://pubs.geoscienceworld.org/jgs/article-pdf/144/2/281/4888788/gsjgs.144.2.0281.pdf by guest on 02 October 2021 282 S. M. WICKHAM effective viscosity at crystal volume fractions in the 50-70% range, where the physical properties of the magma begin to be controlled by theproperties of the solid components ratherthan by the liquid. This pointmarks the divide between low melt-fraction anatectic rocks studied by migmatite petrologists (gross properties dominated by those of the solid phases), and high melt-fraction granitic magma bodies studied by igneous petrologists and fluid dynamicists (gross properties dominated by those of the liquid). The existence of two distinct areas of research also partly reflects the isolation of mostgranitic plutons fromtheir sourcerocks. Conversely, migmatites can seldom be genetically linked to larger plutons, and many only reflect small-scale partial melting processes occurring at relatively high structural levels in the crust. Deep, lower crustal melting zones,where the voluminous melting required to generate largeplutons is more likely to occur, are rarely exposed and may be hard to identify as such, because most of the magma may have already left the source region. The physical properties of partially molten rock During crustal anatexis, the mechanical properties of rocks change with increasing proportion of melt, from linearly- elastic to elastic-plastic to viscous (Shaw 1965; Bottinga & Weill 1972; Murase & McBirney 1973; Shaw 1980; Knapp & Norton 1981). There will therefore bea variety offlow behaviour from low to high degrees of partial melting. High melt-fractions-magmas as dilute suspensions for various values of R. Also shown are experimental A silicate liquid behavesapproximately as an ideal Newtonian fluid, the viscosity of which depends principally measurements of the relative viscosity of increasingly dense suspensions, compiled by Thomas (1965). Figure 1 is thus a on the chemical composition of the melt, and the pressure compilation of experimental data and empirical curves and temperature (Bottinga & Weill 1972). The viscosities of common silicate liquids are well knownfrom experiments fitted to experimental data; thesecurves all suggest little change in the effective viscosity of suspensions for 0.25. (e.g. Shaw 1965; Bottinga & Weill 1972; Murase & < McBirney 1973) and cancalculatedbe easily from This implies that granitic magma bodies withlow crystal compositional data using empirical models (e.g. Shaw 1972). contents (<25%) will have similar viscosities to the same Dueto variablemagmatic water content and to a lesser crystal-free liquids. Furthermore,the viscosityis not extent, temperature, granitic magmas show a wide range in increased by much more than an order of magnitude when = 0.5 (analogous to a half-crystallized magma). viscosity, ranging from 10’ Pa S to 101*Pa S. If a magma contains suspended crystals, it can be treated assuspension,a with certain effective fluid properties Magmas as dense suspensions (Jeffrey & Acrivos 1976; Wildemuth & Williams 1984). In addition to particlevolume fraction, numerous extra Suspensions may be simply modelledas Newtonian fluids factors influence the effective viscosity of dense suspensions, with effective viscosity dependent solely onthe melt resulting in non-Newtonian macroscopic behaviour, (Jeffrey viscosity and the fraction of suspended solids (e.g. Roscoe & Acrivos 1976; McBirney & Murase 1984). Among the 1953; Shaw 1965; Arzi 1978), although many other factors more important factorsrelevant to geological systems are such as the size, shape and distribution of the particles, and graininteractions involving the
Details
-
File Typepdf
-
Upload Time-
-
Content LanguagesEnglish
-
Upload UserAnonymous/Not logged-in
-
File Pages17 Page
-
File Size-