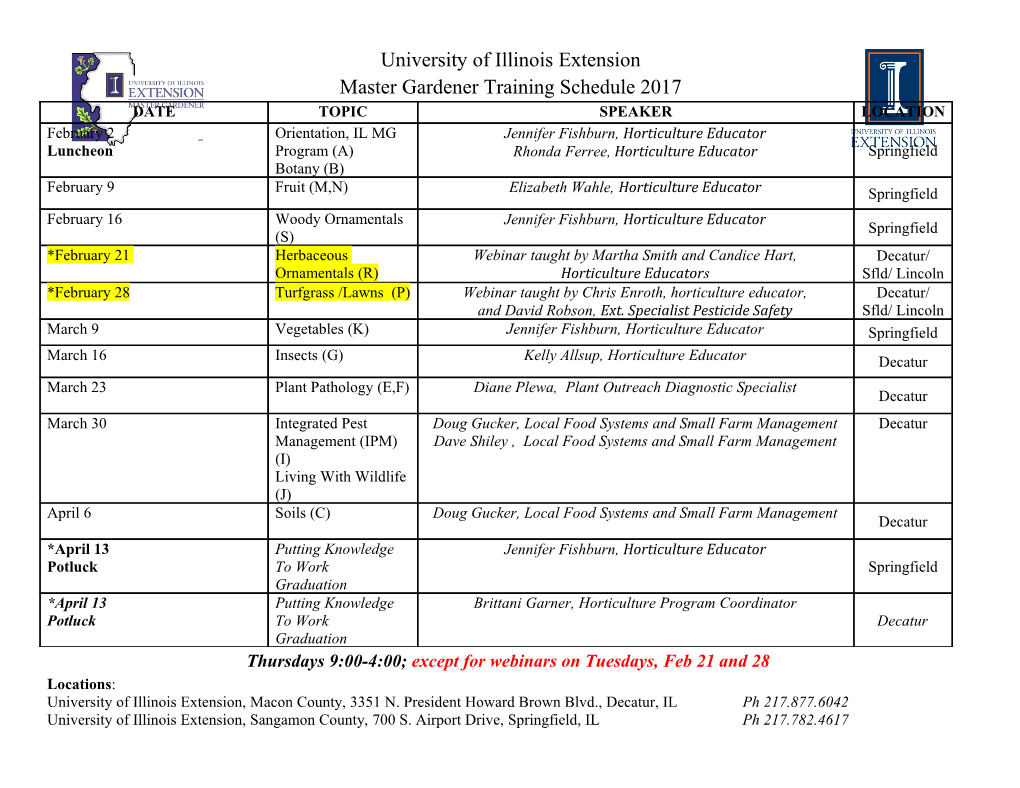
82 Dedifferentiation, Transdifferentiation, and Reprogramming: Future Directions in Regenerative Medicine Cristina Eguizabal, PhD1 Nuria Montserrat, PhD1 Anna Veiga, PhD1,2 Juan Carlos Izpisua Belmonte, PhD1,3 1 Center for Regenerative Medicine in Barcelona Address for correspondence and reprint requests Juan Carlos Izpisua 2 Reproductive Medicine Service, Institut Universitari Dexeus, Belmonte, PhD, Gene Expression Laboratory, The Salk Institute for Barcelona, Spain Biological Studies, 10010 North Torrey Pines Road, La Jolla, CA 93027 3 Gene Expression Laboratory, The Salk Institute for Biological Studies, (e-mail: [email protected]). La Jolla, California Semin Reprod Med 2013;31:82–94 Abstract The main goal of regenerative medicine is to replace damaged tissue. To do this it is Keywords necessary to understand in detail the whole regeneration process including differenti- ► regenerative ated cells that can be converted into progenitor cells (dedifferentiation), cells that can medicine switch into another cell type (transdifferentiation), and somatic cells that can be ► stem cells induced to become pluripotent cells (reprogramming). By studying the regenerative ► dedifferentiation processes in both nonmammal and mammal models, natural or artificial processes ► transdifferentiation could underscore the molecular and cellular mechanisms behind these phenomena and ► reprogramming be used to create future regenerative strategies for humans. To understand any regenerative system, it is crucial to find the potency and differentiate and how they can revert to pluri- cellular origins of renewed tissues. Using techniques like potency (reprogramming) or switch lineages (dedifferentia- genetic lineage tracing and single-cell transplantation helps tion and transdifferentiation). to identify the route of regenerative sources. These tools were We synthesize the studies of different model systems to developed first in nonmammal models (flies, amphibians, and highlight recent insights that are integrating the field. Where- fish) and then in mammal models like mice. The source of cells as previous reviews largely focused on specific animal mod- during regeneration in most cases is either stem cells or els, molecular pathways, or only on one regenerative route, progenitor cells, or the dedifferentiated or transdifferentiated our aim is to combine the three regenerative routes in several cells within the tissue of origin. Another process related to species and discuss future directions in regenerative regenerative therapies is reprogramming: somatic cells that medicine. can be converted into stem cells, known as induced pluripo- fi tent stem cells (iPSs). These arti cial stem cells are suitable for Dedifferentiation several purposes: basic research, drug screening, disease modeling, or autologous cell therapy. Dedifferentiation is the reverse developmental process in This document was downloaded for personal use only. Unauthorized distribution is strictly prohibited. The promising future of regenerative medicine is to re- which differentiated cells with specialized functions become place or regenerate tissues or organs to restore or reestablish undifferentiated progenitor cells. Dedifferentiation and sub- normal cell function. To do so, it is necessary to understand sequent proliferation provide the basis for tissue regeneration the three regenerative processes: dedifferentiation, trans- and the formation of new stem cell lineages. differentiation, and reprogramming. By using the model developed by Waddington1,2 (►Fig. 1), it is possible to In Vivo Dedifferentiation understand the epigenetic status and the developmental Historically, the first evidence of dedifferentiation during the potential of each cell during these processes. This modified regeneration process was found in plants.3 Basically, it can be schematic representation explains graphically how cells lose divided into different types: the regeneration of a tissue Issue Theme Stem Cells Helping Copyright © 2013 by Thieme Medical DOI http://dx.doi.org/ Reproductive Medicine; Guest Editor, Publishers, Inc., 333 Seventh Avenue, 10.1055/s-0032-1331802. Carlos Simón, MD, PhD New York, NY 10001, USA. ISSN 1526-8004. Tel: +1(212) 584-4662. Future Directions in Regenerative Medicine Eguizabal et al. 83 Figure 1 Dedifferentiation, transdifferentiation, and reprogramming processes in Waddington’s epigenetic landscape shows cell populations with different epigenetic and developmental potentials. A modification of the original and modified Waddington’s landscape.1,2 EG cells, embryonic germ cells; EC cells, embryonic carcinoma cells; ICM/ES cells, inner cell mass/embryonic stem cells; iPS cells, induced pluripotent stem cells; mGS cells, multipotent germline stem cells. structure lost after an injury, the de novo generation of a new lineage analysis revealed that the regenerating cells main- tissue structure not present before the injury, and the regen- taining the memory of the earlier cellular identity give rise to eration of the whole plant from a single somatic cell.4 Recent tissues only within their original lineage.15 A recent contro- studies on the generation of callus have shown that it versial study in Xenopus limb regeneration confirmed the regenerates due to a preexisting pluripotent stem cell popu- important role of SALL4 in the dedifferentiation and mainte- lation instead of a dedifferentiation process.5 More studies nance of the blastema cells in an undifferentiated state. More are still needed to evaluate this discrepancy. studies on limb regeneration are needed to elucidate the real In nonmammalian vertebrates, there are several examples mechanisms involved in limb regeneration in amphibians. of dedifferentiation; one of them is bone regeneration via In the case of tail regeneration in the axolotl, tracking dedifferentiation of the osteoblast in zebrafish fin.6 Knopf and fluorescent-labeled single muscle fibers and observing the colleagues monitored osteoblast differentiation in the regen- mononuclear state of muscle fiber cells as a “less differentiat- erating fin using a transgenic system expressing fluorescent ed” state, muscle cells confirmed the dedifferentiation of proteins under the control of promoters of early, intermedi- mature fibers. In the same manner, authors observed an ate, and late osteoblast-specific genes (RUNX2, SP7, and BGLAP increase of the transcription factor MSX-1, known to be – [osteocalcin]).7 9 Their elegant study showed that in a re- expressed in early development during epithelial-mesenchy- sponse to the amputation, mature osteoblasts dedifferentiate, mal transition. Taken together, all of this provides evidence become proliferative, and migrate distally to form outer that the terminally differentiated myotubes dedifferentiated – regions of the regeneration of blastema of fin rays. toward multipotent cells.16 19 Another example of dedifferentiation occurs during heart The dedifferentiation process has been shown to be related regeneration in zebrafish. Jopling and colleagues10 described to the entry of the regenerating cells into the cell cycle. It has that zebrafish heart can fully regenerate up to 20% after been observed that during regenerative dedifferentiation, the – This document was downloaded for personal use only. Unauthorized distribution is strictly prohibited. amputation in the ventricle.11 13 To explain these data, the tumor suppressor retinoblastoma protein (RB) plays a key authors generated induced green fluorescence protein (GFP) role in the reentry of the cells into cell cycle. Along these lines, transgenic zebrafish under cardiomyocyte-specificpro- it has been shown that Drosophila melanogaster mutants for moters (mlc2a and gata4). During this regeneration process, RB and Hippo (a member of the STE20 family of protein fully differentiated cardiomyocytes can dedifferentiate and kinases) maintain a normal neuronal differentiation program. proliferate, regenerating the missing part of the ventricle.10,14 However, the cells do not retain their differentiated status. GFP-labeled cells indicated that the newly generated cardio- They dedifferentiate to an earlier eye-precursor stage show- myocytes originated from existing GFP cardiomyocytes and ing unrestricted proliferation. So far, it seems that dediffer- not from a source of progenitors. entiation and the cell cycle processes are distinct from one Another good example of dedifferentiation is limb regen- another and that RB also plays an important role in main- eration in urodeles (salamander). At first the blastema (group taining the differentiated status of the cell.20,21 of cells that initiates the regenerative process) was regarded In mammals, the capacity of regeneration following the as a homogeneous cell population, but it was later demon- dedifferentiation strategy is limited. However, it has been strated to be a heterogeneous cell population containing observed that in the mouse model, Schwann cells possess the progenitor cells with restricted potential. A specificcell natural ability to regenerate. During development, Schwann Seminars in Reproductive Medicine Vol. 31 No. 1/2013 84 Future Directions in Regenerative Medicine Eguizabal et al. Figure 2 In vivo and in vitro dedifferentiation in mammals. (A) Schwann precursors dedifferentiate toward immature Schwann cells and then finally differentiate into mature myelinating or nonmyelinating Schwann cells. (B) Human tyroid follicular cells can be dedifferentiated into multilineage progenitor cells by culturing them in serum-free conditions. (C) Human epidermal
Details
-
File Typepdf
-
Upload Time-
-
Content LanguagesEnglish
-
Upload UserAnonymous/Not logged-in
-
File Pages13 Page
-
File Size-