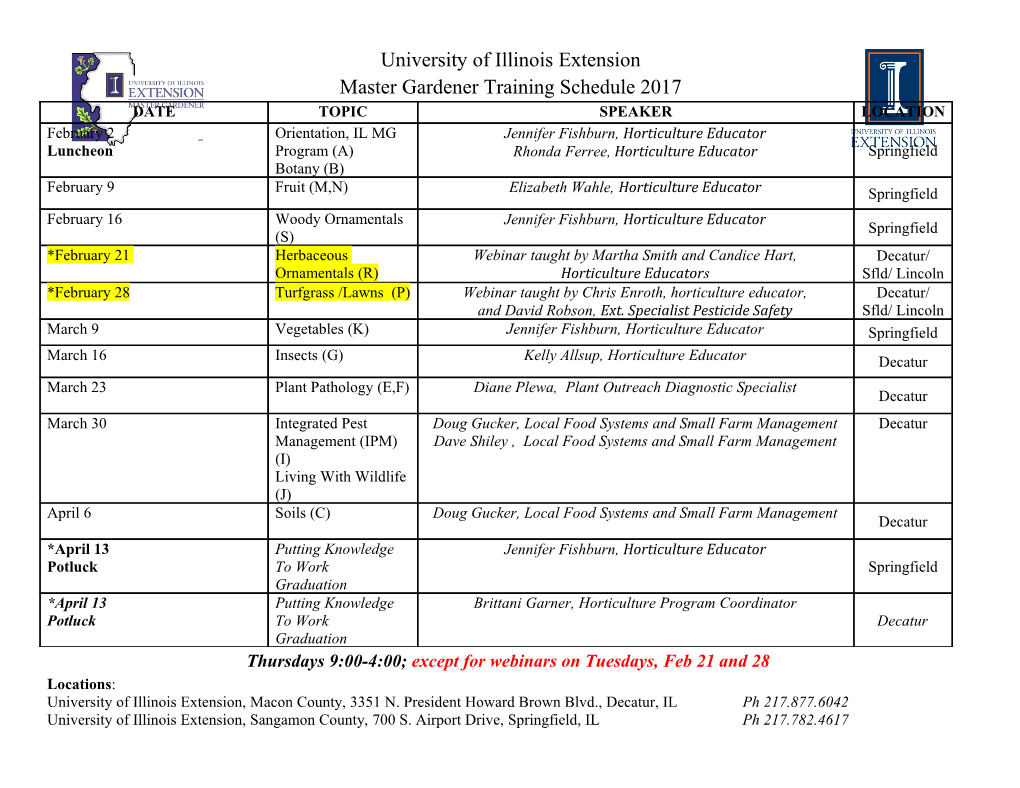
Scanning Confocal Microscopy with A Microlens Array Antony Orth*, and Kenneth Crozier y School of Engineering and Applied Sciences, Harvard University, Cambridge, Massachusetts 02138, USA Corresponding authors: * [email protected], y [email protected] Abstract: Scanning confocal fluorescence microscopy is performed with a refractive microlens array. We simul- taneously obtain an array of 3000 20µm x 20µm images with a lateral resolution of 645nm and observe low power optical sectioning. OCIS codes: (180.0180) Microscopy; (180.1790) Confocal microscopy; (350.3950) Micro-optics High throughput fluorescence imaging of cells and tissues is an indispensable tool for biological research[1]. Even low-resolution imaging of fluorescently labelled cells can yield important information that is not resolvable in traditional flow cytometry[2]. Commercial systems typically raster scan a well plate under a microscope objective to generate a large field of view (FOV). In practice, the process of scanning and refocusing limits the speed of this approach to one FOV per second[3]. We demonstrate an imaging modality that has the potential to speed up fluorescent imaging over a large field of view, while taking advantage of the background rejection inherent in confocal imaging. Figure 1: a) Experimental setup. Inset: Microscope photograph of an 8x8 sub-section of the microlens array. Scale bar is 80µm. b) A subset of the 3000, 20µm x 20µm fields of view, each acquired by a separate microlens. Inset: Zoom-in of one field of view showing a pile of 2µm beads. The experimental geometry is shown in Figure 1. A collimated laser beam (5mW output power, λex =532nm) is focused into a focal spot array on the fluorescent sample by a refractive microlens array. The 100x100 element microlens array is fabricated using a reflow molding technique[4], and replica molded into Norland Optical Adhesive (NOA) 61 (n ≈ 1:56 at 532nm)[5]. The microlenses have a diameter of 37µm, height of 15µm and pitch of 55µm. The microlenses create a focal spot with FWHM = 740nm as imaged by a 50x 0.55 NA objective lens. The focal length of the microlenses is measured to be 37µm, giving an NA of 0.44. Assuming the microlenses take on a spherical profile, their large curvature leads to spherical aberration due to over-refraction of rays near the periphery of the microlens[4]. Spherical aberration is apparent in an axial slice of a 1µm bead shown in Figure 2 b). Fluorescence excited at each focal spot is collected by its respective microlens, ie. each microlens is detecting signal in an epi-fluorescent geometry. The collected fluorescence (λem =575nm) is collimated by each microlens, passes through a dichroic mirror and is imaged onto a CCD camera by an f = 75mm focal length relay lens. The relay lens is placed 2f behind the microlens array and 2f in front of the CCD camera. A 3mm diameter iris is placed at a distance f behind the relay lens in order to confocally filter the fluorescence emission from each microlens[6]. A typical image relayed to the CCD is shown in Figure 1 b). The brightness of the back aperture of each microlens corresponds to the fluorescence intensity excited by the local focal spot. The sample is raster scanned over a 20µm 1 x 20µm area using a closed-loop piezo stage (field size limited by the piezo travel), with a step size of 175nm. For each microlens, a 20µm x 20µm image is formed by summing the pixel values in the image of the back aperture of the microlens at each scan position, and assigning this value to the appropriate pixel in the 20µm x 20µm field. The fluorescence relayed by the microlenses is recorded by the CCD camera at 202 frames per second (fps), and the piezo raster scan is set to have an integral number of camera frames per line to avoid image shearing. Fluorescent microspheres of various sizes are dried onto a microscope slide for imaging. An image of multiple fields of view scanned simultaneously by the microlens array is shown in Figure 1 b). Due to laser power limitations, only ∼3000 lenses are illuminated. Figures 2 c) and d) show zoomed-in portions of two FOVs containing clumps of 2µm and 5µm fluorescent microspheres. From a Gaussian fit to the cross section of a 500nm bead (Figure 2 a)), we obtain a lateral FWHM of 645nm, equivalent to the resolving power of a NAc=0.37 confocal microscope[7]. We also observe low power optical sectioning. Figure 2 b) shows an axial slice of a 1µm diameter fluorescent bead. The FWHM of the bead along the optical axis is extended to 3.75µm, equal to that expected of a NAc=0.45 confocal microscope. The axial section also displays a long tail, indicative of the spherical aberration present in the microlenses. Figure 2: a) Cross section of 500nm fluorescent bead with a Gaussian fit denoted by the dotted line. Inset: Image of 500nm fluorescent bead used for fitting. b) Axial slice of a 1µm fluorescent bead, FWHM=3.75µm as measured by a Gaussian fit. c) and d) Piles of 2 and 5µm beads. Despite the slow scanning employed here (1.5 lines per second), a relatively high pixel throughput of 0.6 Megapix- els/s is maintained by point scanning 3000 FOVs at 202 fps. The pixel throughput is limited by the camera speed, and can be improved by several orders of magnitude by sampling faster in time and by using a larger CCD area. At max- imum pixel throughput, we estimate that the MotionXtra N-4 camera (Redlake) can reach a useable pixel throughput of 123 Megapixels/s[8]. By comparison, a microplate reader takes on average 1s per field of view, yielding on the order of 1-10 Megapixels/s[3]. Piezo scanning at these high speeds is possible using feedforward algorithms [9]. In addition to the piezo scanning geometry described here, we expect our imaging technique to be applicable to integrated microscopy in microfluidics[10]. Future work is ongoing in this area. References [1] R. Pepperkok and Jan Ellenberg, “High-throughput fluorescence microscopy for systems biology," Nature Reviews Molecular Cell Biology 7, 690-696 (2006). [2] D. A. Basij et al., “Cellular Image Analysis and Imaging by Flow Cytometry," Clinics in Laboratory Medicine 3, 653-670 (2007). [3] Olympus ScanR Website. http://www.microscopy.olympus.eu/microscopes/Life_Science_Microscopes_scan_R_Specifications.htm#Performance. Accessed 20 May 2011. [4] D. Daly, R. F. Stevens, M. C. Hutley and N. Davies, “The manufacture of microlenses by melting photoresist", Meas. Sci. Technol. 1, 759-766 (1990). [5] Norland Optical Adhesive 61 Data Sheet. http://www.norlandprod.com/adhesives/noa61pg2.html. Accessed 20 May 2011. [6] H. J. Tiziani and Hans-Martin Uhde, “Three-dimensional analysis by a micro lens-array confocal arrangement", Applied Optics 33, 567-572 (1994). [7] M. Mueller, Introduction to Confocal Fluorescence Microscopy, SPIE Press (2005). [8] Camera specification sheet, Redlake Distributor Website. http://www.idtvision.com/imaging/n4.php?show=1. Accessed 20 May 2011. [9] Y. Li and J. Bechhoefer,“Feedforward control of a closed-loop piezoelectric translation stage for atomic force microscope" Rev. Sci. Inst. 78, 013702 (2007). [10] G. Zheng et al.,“Sub-pixel resolving optoSSuidic microscope for on-chip cell imaging" Lab Chip 10, 3125-3129 (2010). 2.
Details
-
File Typepdf
-
Upload Time-
-
Content LanguagesEnglish
-
Upload UserAnonymous/Not logged-in
-
File Pages2 Page
-
File Size-