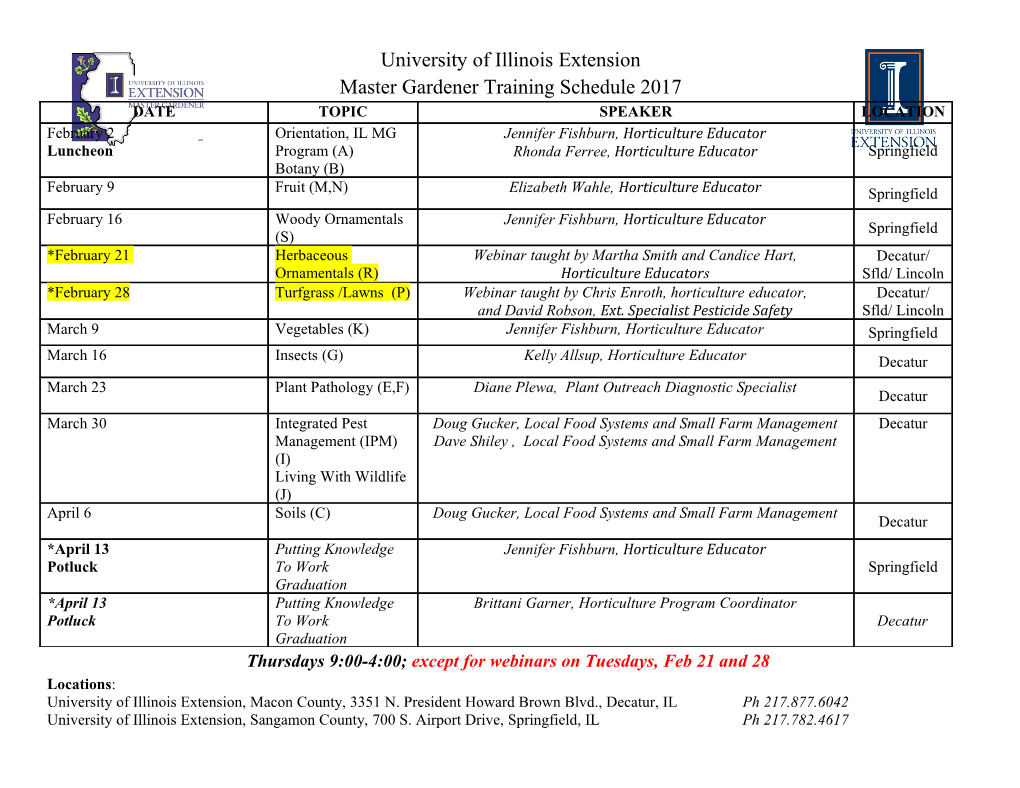
Heteroleptic actinocenes: a thorium(iv)- cyclobutadienyl-cyclooctatetraenyl-di- potassium-cyclooctatetraenyl complex. Item Type article Authors Boronski, Josef T; orcid: 0000-0002-1435-6337; Wooles, Ashley J; orcid: 0000-0001-7411-9627; Liddle, Stephen T; orcid: 0000-0001-9911-8778 Citation Chemical science, volume 11, issue 26, page 6789-6794 Rights Licence for this article: cc by Download date 28/09/2021 16:00:53 Link to Item http://hdl.handle.net/10034/625194 Chemical Science EDGE ARTICLE Heteroleptic actinocenes: a thorium(IV)– cyclobutadienyl–cyclooctatetraenyl–di- Cite this: Chem. Sci., 2020, 11,6789 potassium-cyclooctatetraenyl complex† All publication charges for this article have been paid for by the Royal Society of Chemistry Josef T. Boronski, Ashley J. Wooles and Stephen T. Liddle * n Despite the vast array of h -carbocyclic C5–8 complexes reported for actinides, cyclobutadienyl (C4) remain exceedingly rare, being restricted to six uranium examples. Here, overcoming the inherent challenges of installing highly reducing C4-ligands onto actinides when using polar starting materials such as halides, 8 4 8 we report that reaction of [Th(h -C8H8)2] with [K2{C4(SiMe3)4}] gives [{Th(h -C4[SiMe3]4)(m-h -C8H8)(m- 2 h -C8H8)(K[C6H5Me]2)}2{K(C6H5Me)}{K}] (1), a new type of heteroleptic actinocene. Quantum chemical calculations suggest that the thorium ion engages in p- and d-bonding to the h4-cyclobutadienyl and h8-cyclooctatetraenyl ligands, respectively. Furthermore, the coordination sphere of this bent thorocene Received 1st May 2020 analogue is supplemented by an h2-cyclooctatetraenyl interaction, which calculations suggest is Accepted 10th June 2020 composed of s- and p-symmetry donations from in-plane in- and out-of-phase C]C 2p-orbital DOI: 10.1039/d0sc02479a combinations to vacant thorium 6d orbitals. The characterisation data are consistent with this being rsc.li/chemical-science a metal–alkene-type interaction that is integral to the bent structure and stability of this complex. Introduction elements. Indeed, although transition metal cyclobutadienyl chemistry was established in the 1960s,5 the rst f-element- 8 The synthesis of uranocene, [U(h -C8H8)2], the rst actinocene, cyclobutadienyl complex, an inverted sandwich tetraphenylcy- 8 6,7 was reported in 1968, and its cousin thorocene, [Th(h -C8H8)2], clobutadienyl diuranium(IV) species, was reported in 2013. emerged in 1969.1 Uranocene was a landmark discovery, not Very recently, the uranium(IV)–cyclobutadienyl half sandwich only in the eld of f-element chemistry, but organometallic pianostool complex [U{C4(SiMe3)4}(BH4)3][Li(THF)4](A) was re- chemistry as a whole, and it inspired research into the degree of ported,8 and shortly aer that four uranium(IV)–cyclobutadienyl 9 5f/6d orbital participation in metal–ligand bonding that is still complexes [U{C4(SiMe3)4}(BH4)3][Na(12-crown-4)], [U{C4- 2 m 9 k3 burgeoning today. Furthermore, due to the steric and elec- (SiMe3)4}(BH4)2( -BH4){K(THF)2}]2, [U(BH4){C4(SiMe3)4}{ - k t 9 tronic versatility of cyclooctatetraenyl dianions, which provide C4H(SiMe3)3- -(CH2SiMe2)}][Na( BuOMe)3.6(THF)0.4], and m m 10 four-fold symmetry bonding combinations with 5f/6d orbitals, [{U(C4[SiMe3]4)( -I)2}3{ 3-O}][Mg(THF)6] were disclosed. These this ligand class has been used widely in organoactinide six uranium–cyclobutadienyl complexes constitute all actinide– chemistry,2d,3 with uranium- and thorium–cyclooctatetraenyl cyclobutadienyl chemistry to date, in stark contrast to the hn 11 complexes being found to exhibit uncommon bonding, oxida- plethora of reported f-element -carbocyclic C5–9 complexes. tion state, and ligand motifs.4 Thus, a thorium–cyclobutadienyl complex of any kind is Despite advances in actinide science enabled by cyclo- conspicuous by its absence, but realising such a target would octatetraenyl ligands, the closely-related, but far smaller, dia- provide comparisons between uranium and thorium and nionic cyclobutadienyl ligand, which also provides up to four- provide further insight into actinide–cyclobutadienyl bonding. fold symmetry bonding combinations to metals, has, thus far, Herein, we report the synthesis of the rst thorium–cyclo- remained barely investigated. This possibly reects the paucity butadienyl complex, which, containing cyclobutadienyl and of suitable starting materials and the proclivity of cyclo- cyclooctatetraenyl ligands, is an unprecedented heteroleptic butadienyls to decompose via reductive, protonolysis, or cyclo- actinocene analogue. The thorium ion engages in p- and d- metallation routes when in the coordination sphere of polar f- bonding to the cyclobutadienyl and cyclooctatetraenyl ligands, respectively, and the coordination sphere of this bent thorocene analogue is supplemented by an h2-cyclooctatetraenyl interac- Department of Chemistry, The University of Manchester, Oxford Road, Manchester, tion from co-complexed dipotassium-cyclooctatetraenyl. M13 9PL, UK. E-mail: [email protected] Quantum chemical studies reveal that this thorium–h2-cyclo- † Electronic supplementary information (ESI) available: Synthetic, structural, octatetraenyl interaction is composed of s- and p-symmetry spectroscopic, and computational details. CCDC 1992448 (1). For ESI and ] crystallographic data in CIF or other electronic format see DOI: alkene-like donation from in-plane in- and out-of-phase C C 10.1039/d0sc02479a 2p-orbital combinations to formally vacant thorium 6d orbitals. This journal is © The Royal Society of Chemistry 2020 Chem. Sci.,2020,11,6789–6794 | 6789 Chemical Science Edge Article Results and discussion vacuum, but they do exchange with benzene when 1 is dissolved in this solvent. Synthetic considerations We previously reported that reaction of [Li2{C4(SiMe3)4}(- Crystallographic characterisation THF) ]12 with UCl led to reduction of uranium(IV) to intractable 2 4 The solid-state structure of 1 was determined by X-ray diffrac- mixtures of uranium(III)-containing products.8 However, thor- tion (Fig. 1). Complex 1 crystallises with two [Th{h4- q / ium(IV) has a far greater reduction potential (E Th(IV) Th(III) m h8 m h2 q C4(SiMe3)4}( - -C8H8)( - -C8H8)(K)2] units in the crystallo- À3.7 V) than that of uranium(IV)(E U(IV) / U(III) À0.6 V).13 graphic asymmetric unit, rendered inequivalent to each other Nevertheless, addition of a solution of [K {C (SiMe ) }]14 to 2 4 3 4 by the nature of K-coordination environments: K4-(h6- 15 [ThCl4(THF)3.5] resulted in oxidation of dianionic h8 h2 h6 h1 h8 À C6H5Me)( -C8H8)( -C8H8); K3-( -C6H5Me)( -C6H5Me)( - {C (SiMe ) }2 to the neutral cyclobutadiene {C (SiMe ) },16 as 4 3 4 4 3 4 C H ); K2-(h6-C H Me)(h6-C H Me)(h8-C H ); K1-(h8-C H )(h2- 8 8 6 5 6 5 8 8 8 8 con rmed by multinuclear NMR spectroscopy, and deposition k1 C8H8)( -MeSiMe2). The latter interactions double-up the of an insoluble dark grey precipitate presumed to be KCl and asymmetric unit resulting in a tetrathorium aggregate overall.17 colloidal thorium.17 This underscores the strongly reducing 2À In more detail, the salient structural features of each thorium- nature of {C4(SiMe3)4} and led us to conclude that polar 4 2À containing unit are h -coordination of the {C4(SiMe3)4} dia- actinide halides are unsuitable starting materials for our 2À 8 2 nion and two {C8H8} ligands which are h -and h -coordi- purposes. Advancing an alternate strategy, it was reasoned that 2À nated, the latter of which is highly unusual for a {C8H8} less polar thorium(IV)–carbocyclic complexes would be less ligand. Thus, the coordination geometry at each thorium ion prone to the undesirable redox chemistry observed for actinide- resembles that of a bent C4/C8-metallocene with a coordinated halides. On hard-so acid-base theory grounds, [Th(h8-C H ) ] 8 8 2 alkene, the latter being a rare interaction for lanthanides19 and was selected as a starting material1,18 since we anticipated that À unknown in actinide chemistry, with the closest example for displacement of the so, charge-diffuse 10p-{C H }2 dianion 8 8 actinides being the reduced 1,2-ethanediide [{(h8-C H [- À p 8 4 (1.25e per carbon) by the hard, charge-concentrated 6 - i h5 m h2 h2 20 2À À SiPr 3]2)( -C5Me5)U}2( - - -C2H4)]. {C4(SiMe3)4} dianion (1.5e per carbon) would be favourable 4 The average Th–C(h -C4(SiMe3)4) distances are 2.651(9) and for the hard thorium(IV) ion. 2.649(9) A for Th1 and Th2, respectively; 0.14 A greater than Condensation of THF onto a cold (À196 C) solid mixture of analogous value found for A (2.513(17) A), though when [Th(h8-C H ) ] and [K {C (SiMe ) }], followed by thawing and 8 8 2 2 4 3 4 considering the 3s-criterion this difference falls to 0.06 A.8 The heating for two hours, led to the formation of a bright orange latter is close to the 0.05 Adifference in the ionic six-coordinate ff solution. A er work-up, recrystallisation from toluene a orded 21 radii of thorium(IV) (0.94 A) and uranium(IV) (0.89 A). Inter- bright orange crystals, the colour of which assures the presence – h4 ff 4 estingly, the Th C( -C4(SiMe3)4) distances span quite di erent of thorium(IV), formulated as the co-complex [{Th(h - m h8 m h2 C4[SiMe3]4)( - -C8H8)( - -C8H8)(K[C6H5Me]2)}2{K(C6H5Me)} {K}] (1) in 78% isolated yield (Scheme 1).17 Interestingly, 1 does not appear to react further if an additional equivalent of [K2{C4(SiMe3)4}] is added. The coordinated toluene molecules in 1 do not de-coordinate when 1 is placed under dynamic Fig.
Details
-
File Typepdf
-
Upload Time-
-
Content LanguagesEnglish
-
Upload UserAnonymous/Not logged-in
-
File Pages7 Page
-
File Size-