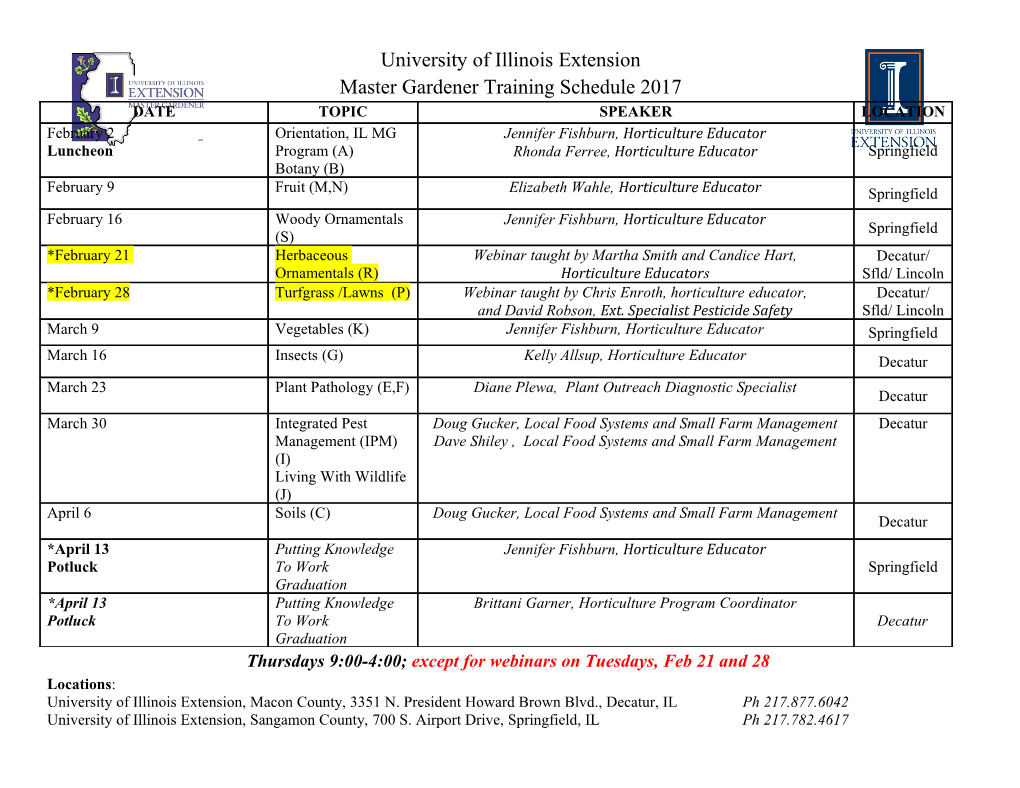
Imperial College London Department of Physics Physics and Computational Simulations of Plasma Burn-through for Tokamak Start-up Hyun-Tae Kim Submitted in part fulfilment of the requirements for the degree of Doctor of Philosophy in Physics of Imperial College London, July 2013 Abstract This thesis will discuss the fundamental process of high temperature plasma formation, con- sisting of the Townsend avalanche phase and the subsequent plasma burn-through phase. By means of the applied electric field, the gas is partially ionized by the avalanche process. In order for the electron temperature to increase, the remaining neutrals need to be fully ionized in the plasma burn-through phase, as radiation is the main contribution to the electron power loss. The radiated power loss can be significantly affected by impurities resulting from inter- action with the plasma facing components. The parallel transport to the surrounding walls is determined by the so called connection length in the plasma. Previously, plasma burn-through was simulated with the assumptions of constant particle con- finement time and impurity fraction. In the new plasma burn-through simulator, called the DYON code, the treatment of particle confinement time is improved with a transonic ambipo- lar model for parallel transport, by using the effective connection length determined by the magnetic field lines, and Bohm diffusion model for perpendicular transport. In addition, the dynamic evolution of impurity content is calculated in a self-consistent way, using plasma wall interaction models. The recycling of the particles at the walls is also modelled. For a specific application, the recent installation of a beryllium wall at Joint European Torus (JET) enabled to investigate the effects of plasma facing components on plasma formation and build-up of plasma current in the device. According to the JET experiments the Townsend avalanche phase was not influenced by the replacement of the wall material. However, failures during the plasma burn-through phase, that could occur with a carbon wall, are not observed with a beryllium wall. In order to obtain a deeper insight in these effects this thesis will present detailed modelling of plasma burn-through. For the first time a quantitative validation of the simulation results to experimental data is documented. The simulation results with the DYON code show consistent good agreement against JET data obtained with the carbon wall as well as the beryllium wall. According to the DYON results, the radiation barrier in a carbon wall is dominated by the carbon radiation. The radiation barrier in the beryllium wall is mainly from the deuterium radiation rather than the beryllium radiation, as far as the radiated power from other impurities (i.e. carbon, nitrogen, etc) is not significant. These issues are of crucial importance for the International i Thermonuclear Experimental Reactor (ITER) where the allowable toroidal electric field for plasma formation is limited to 0.35 V/m, which is significantly lower compared to the typical loop voltage ( 1 V/m) used in the current devices. Using the validated DYON code, predictive simulations for ITER are given, showing a need for RF heating to allow reliable plasma burn- through. ii Supervisors and Examiners This thesis has been completed under supervision of • Dr A.C.C. Sips, EFDA-CSU, Culham Science Centre, OX14 3DB, Abingdon, UK • Dr Wojtek Fundamenski, Department of Physics, Imperial College London, SW7 2AZ, London, UK • Prof Steven Rose, Department of Physics, Imperial College London, SW7 2AZ, London, UK Thesis examination commitee: • Dr Brian Lloyd, EURATOM/CCFE, Culham Science Centre, OX14 3DB, Abingdon, UK • Dr Robert Kingham, Department of Physics, Imperial College London, SW7 2AZ, Lon- don, UK iii iv Acknowledgements First of all, I would like to thank my supervisor, Dr. A.C.C. Sips. He made enormous contri- bution to this thesis. His insightful comments, warm encouragement, and active support were invaluable. I would also like to thank my previous supervisor, Dr. Wojtek Fundamenski, who gave me a PhD opportunity and great supervision for the first two years. Also, I appreciate Prof. Steve Rose for allowing me to have generous support from Imperial College. I am grateful to Dr. Peter de Vries for being a great academic adviser in Culham. Discussions with him have been very illuminating. I would like to express my gratitude to Kwan-Jeong education foundation for their financial support. Also, I would like to thank Prof. Sang-Hee Hong and Prof. Yong-Seok Hwang who first taught me Plasma Physics, and Prof. Oh-Jin Kwon who helped me to find a PhD opportunity in the UK. I owe a very important debt to my parents-in-law, who have been looking after DYON. Without their devotion, I might not have been able to continue my PhD study. I also wish to thank my parents, grandmothers, and younger brother, who always pray for me. Finally, I want to express the deepest appreciation to my wife, Jay-Young. Special thanks to her for the great time we have had together. This thesis would not have been possible without her heartfelt encouragement and persistent help. v vi Dedication I confirm that the work presented in this thesis is entirely my own, except where indicated by references in the main text. Hyun-Tae Kim July 2013 vii viii Copyright Declaration The copyright of this thesis rests with the author and is made available under a Creative Commons Attribution Non-Commercial No Derivatives licence. Researchers are free to copy, distribute or transmit the thesis on the condition that they attribute it, that they do not use it for commercial purposes and that they do not alter, transform or build upon it. For any reuse or redistribution, researchers must make clear to others the licence terms of this work. ix x List of Publications The journal papers related to the thesis work are listed below. 1. Hyun-Tae Kim, Wojtek Fundamenski, A.C.C. Sips, and EFDA-JET contributors, En- hancement of plasma burn-through simulations and validation in JET, Nuclear Fusion 52 103016 (2012) • Included in chapters 3, 5, and 6 in this thesis. 2. Hyun-Tae Kim, A.C.C. Sips, Wojtek Fundamenski, and EFDA-JET contributors, PSI Effects on plasma burn-through in JET, Journal of Nuclear Materials, 438, S1271-S1274 (2013) • Included in chapter 5 in this thesis. 3. Hyun-Tae Kim, A.C.C. Sips, and EFDA-JET contributors, Physics of plasma burn- through and DYON simulations for the ITER-like wall, Nuclear Fusion, 53 083024 (2013) • Included in chapters 4, 5, and 6 in this thesis. 4. Hyun-Tae Kim, A.C.C. Sips, P.C. de Vries, and EFDA-JET contributors, Plasma burn- through simulations using the DYON code and predictions for ITER, Plasma Physics and Controlled Fusion, Accepted (2013) • Included in chapters 7 and 8 in this thesis. xi xii List of Main Conferences The conferences where the thesis work were presented are listed below. 1. (Invited talk) European Physical Society Conference on Plasma Physics, Simulation of plasma formation in magnetized plasmas and the influence of plasma facing components, Espoo, Finland, July 2013 2. IOP Conference on Computational Plasma Physics, Enhancement of plasma burn-through simulation and validation in JET, Brighton, UK, September 2012 3. International Conference on Plasma Surface Interaction, PSI Effects on Plasma Burn- through in JET, Aachen, Germany, May 2012 xiii `We have generated energy from resources, but now we will generate energy from the knowledge.' Steven Cowley, Director of CCFE xiv Contents Abstract i supervisors iii Acknowledgements v Dedication v Copyright Declaration vii List of Publications xi List of main conferences xi 1 Introduction 1 1.1 Nuclear Fusion . 1 1.1.1 Thermonuclear Fusion . 1 1.1.2 Tokamak . 2 1.2 ITER and JET . 8 1.2.1 ITER . 8 1.2.2 JET and ITER-like wall . 10 xv xvi CONTENTS 1.3 Brief explanation of tokamak start-up . 13 1.4 Motivation of plasma burn-through modeling . 18 1.5 Scope of thesis . 19 2 Review of electron avalanche 21 2.1 Introduction . 21 2.2 Townsend avalanche theory . 22 2.2.1 The first townsend coefficient . 22 2.2.2 The second townsend coefficient . 26 2.3 Electron avalanche in tokamaks . 30 2.4 Discussion . 33 3 Plasma burn-through simulation 36 3.1 Introduction . 36 3.2 Basic structure of plasma burn-through simulator . 37 3.2.1 Circuit equation for plasma current . 37 3.2.2 Electron energy balance . 42 3.2.3 Ion energy balance . 45 3.2.4 Particle balance . 46 3.3 Review of the previous models . 48 3.3.1 Confinement time model . 48 3.3.2 Impurity model . 49 3.4 New models for plasma burn-through simulation . 49 3.4.1 Circuit equation for eddy current . 50 CONTENTS xvii 3.4.2 Dynamic effective connection length . 51 3.4.3 Deuterium ion confinement time . 52 3.4.4 Impurity ion transport model . 53 3.4.5 Transonic ambipolar transport of impurity ions . 55 3.4.6 Neutral screening effect deuterium and impurities ............. 57 3.4.7 Impurity particle balance model . 58 3.5 Description of the DYON code . 60 3.6 Discussion . 63 4 Physics of deuterium burn-through 65 4.1 Introduction . 65 4.2 Condition for plasma current ramp-up . 66 4.3 Criterion for deuterium burn-through . 70 4.4 Summary and discussion . 76 5 New models for the carbon wall and the ITER-like wall in JET 77 5.1 Introduction . 77 5.2 Impurity effects on plasma burn-through at JET with carbon wall and ITER-like wall. .......................................... 78 5.2.1 Fractional abundance of impurity in non-coronal equilibrium . 78 5.2.2 Radiation barrier versus impurity influx in JET .
Details
-
File Typepdf
-
Upload Time-
-
Content LanguagesEnglish
-
Upload UserAnonymous/Not logged-in
-
File Pages194 Page
-
File Size-