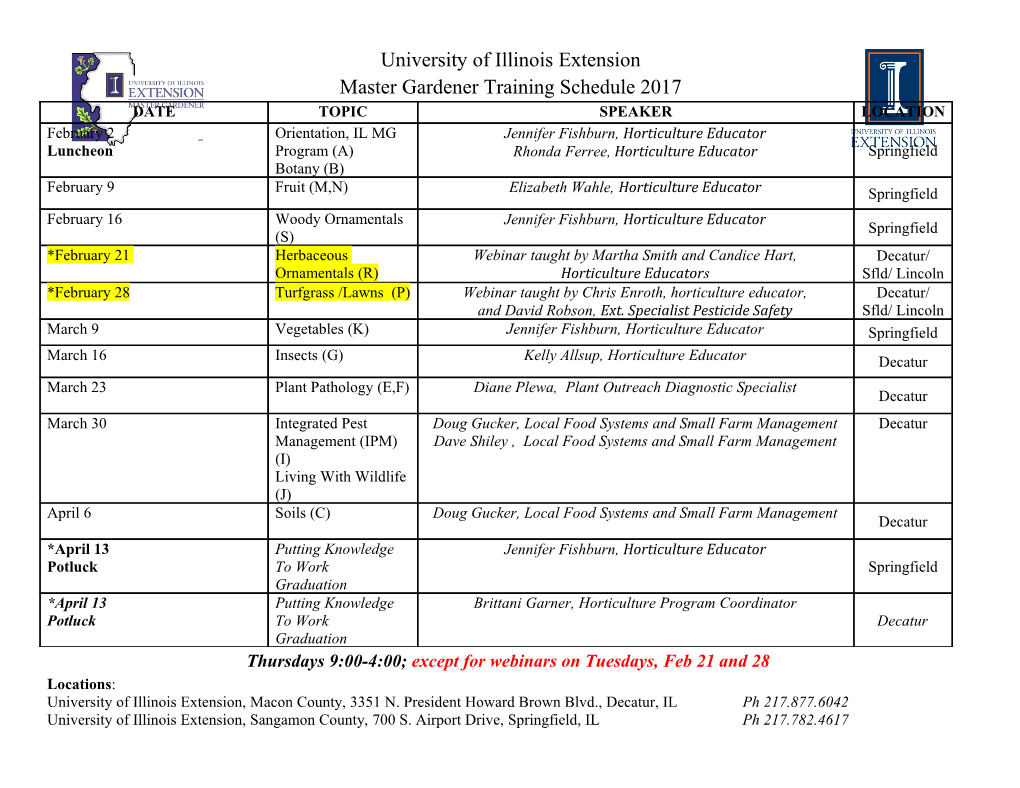
UNIVERSITY OF CALIFORNIA RIVERSIDE The Development of Transition Metal Silylene and Germylene Complexes for Small Molecule Activation A Dissertation submitted in partial satisfaction of the requirements for the degree of Doctor of Philosophy in Chemistry by Marissa Christina Barrientos September 2019 Dissertation Committee: Dr. Hill Harman, Chairperson Dr. Richard Hooley Dr. Yadong Yin Copyright by Marissa Christina Barrientos 2019 The Dissertation of Marissa Christina Barrientos is approved: Committee Chairperson University of California, Riverside Acknowledgments I first and foremost would like to thank my advisor and mentor, Dr Hill Harman. I am forever grateful for the unique experience of being one of your first graduate students. I thank you for all your support and making me the chemist I am now. I would also like to thank the members of my committee, Dr. Richard Hooley and Dr. Yadong Yin. Thank you for all your support and the discussions throughout my time. I would also like to thank the ACIF staff for all their help and support. Thank you to Dr. Dan Borchardt for all your help especially in setting up all those difficult silicon NMR experiments. I would also like to thank Dr. Fook Tham and Dr. Charlene Tsay for their skills and expertise in helping to mount crystals and solving XRD structures. I also want to thank Dr. Richard Kondrat and Dr. Ron New for their expertise in helping to obtain LIFDI results which were invaluable. Last, but certianly not least, I would like to thank Prisciliano Saavedra for all his help in supplying our lab and many solutions to lab issues. I would also like to thank current and former lab members: Dr. Alex McSkimming, Jordan Taylor, Amy Bartrom-Jehl, Laura Essex, Colie Sanchez, Phil Farias, Pranee Pairs, and Andrew Patalano. I would also like to thank my undergraduates that I had the pleasure of mentoring, Daniel Barragan, Joe Mejia, and James Jimenez. I wish to thank members of the Conley group as well for all their support in our joint group meeting ventures. Special thanks to my husband, Robert, for all his support and love through this process. I especially thank him for keeping me sane through this process. Shout-out to my high school chemistry teacher, Mrs. Hamilton, for awakening my love for chemistry iv Last, I give many thanks and gratitude to my parents for their never-ending love while I have pursued a PhD. v To my parents for all the support. vi ABSTRACT OF THE DISSERTATION The Development of Transition Metal Silylene and Germylene Complexes for Small Molecule Activation by Marissa Christina Barrientos Doctor of Philosophy, Graduate Program in Chemistry University of California, Riverside, September 2019 Dr. Hill Harman, Chairperson While N-heterocyclic carbenes (NHC) are widely recognized for their utility as cat- alysts and ligands for catalysts, their heavier analogues, namely N-heterocyclic silylenes and germylenes have not been as extensively explored. These divalent group-14 compounds are intriguing as they have a more accessible empty p-orbital due to decreased overlap with the nitrogen centered lone pairs. This fact results in both nucleophilic and electrophilic charac- ter, or ambiphilicity, in silylenes and germylenes. We are interested in utilizing this prop- erty to design transition metal complexes of N-heterocyclic silylenes (NHSi) and germylenes (NHGe) Herein we report the synthesis of a several diphosphine pincer frameworks anchored by silicon and germanium atoms. In the case of the silicon-based ligands, dichlorosilane, hydrochlorosilane derivatives function as proligands whereas the germanium derivative is accessible as the free germylene. Metallation of the Si-anchored pincers with the Pt group metals (Ni, Pd, and Pt) proceeds by SiCl or SiH activation of the proligands to give divalent square planar complexes featuring a chlorosilyl anchoring ligand. Two-electron reduction of the Pd derivative affords a bimetallic dipalladium(0) disilylene species with inequivalent vii metal sites that are in dynamic exchange in solution. This bimetallic disilylene activates the OH bonds of both water and phenol to give the corresponding disilyl dipalladium(I) species. In contrast to the analogous dinickel system explored in our lab, it does not react with H2. CO2 reacts with the disilylene species to give CO and a bridging disilylcarbon- ate complex. These results highlight the viability of cooperative small molecule activation at late-metal silylene complexes. We also report a large family of bimetallic complexes of first row metals (Mn, Fe, Co, and Ni) supported by the germylene-anchored pincer. Unlike the silicon-based ligands discussed above, which bind metals in a 1:1 ratio, the germylene ligand variant typically binds two metals. These bridging germylene complexes exhibit a range of metal-metal distances and electronic interactions resulting in both diamagnetic and paramagnetic complexes. Unlike the silicon anchored species, the bimetallic germylene complexes were generally electronically and/or coordinatively saturated, and thus typically unreactive.These results highlight the substantial differences in germylene and silylene lig- and owing to the larger size of Ge versus Si. viii Contents List of Figures xi List of Tables xiv List of Schemes xv 1 Introduction 1 1.1 Carbenes . .2 1.2 Silylenes . .5 2 Synthesis of Bidentate Phosphine Ligands 10 2.1 Abstract . 10 2.2 Introduction . 11 2.3 Results and Discussion . 12 2.4 Experimental Section . 14 2.4.1 Synthetic Materials and Methods . 14 t 2.4.2 Cl2Si(NCH2P Bu2)2C6H4 (1)...................... 15 t 2.4.3 HClSi(NCH2P Bu2)2C6H4 (2)...................... 15 t 2.4.4 H2Si(NCH2P Bu2)2C6H4(3)....................... 16 t 2.4.5 Ge(NCH2P Bu2)2C6H4 (4)....................... 17 2.4.6 Ge(NCH2PCy2)2C6H4(5)........................ 17 2.5 References . 18 2.6 Figures, Schemes, and Tables . 19 3 Metallation of the Silylane Framework 34 3.1 Abstract . 34 3.2 Introduction . 35 3.3 Results and Discussion . 36 3.4 Experimental Section . 39 t 3.4.1 NiCl2Si(NCH2P Bu2)2C6H4 (6)..................... 39 t 3.4.2 NiMeBrSi(NCH2P Bu2)2C6H4 (7)................... 40 t 3.4.3 PdCl2Si(NCH2P Bu2)2C6H4 (8).................... 40 ix t 3.4.4 PtCl2Si(NCH2P Bu2)2C6H4 (9)..................... 41 3.5 References . 43 3.6 Figures, Schemes, and Tables . 44 4 Metallation of the Germylene Ligand 54 4.1 Abstract . 54 4.2 Introduction . 55 4.3 Results and Discussion . 56 4.4 Experimental Section . 61 4.4.1 Synthetic Materials and Methods . 61 4.4.2 GeCo2(CO)6(NCH2PCy2)2C6H4 (10)................. 61 4.4.3 GeCo2(CO)5(NCH2PCy2)2C6H4 (11)................. 62 4.4.4 GeFe2(CO)5(NCH2PCy2)2C6H4 (12).................. 62 4.4.5 GeMn2(CO)8(NCH2PCy2)2C6H4 (13)................. 63 4.4.6 GeCo2(C5Me5)2(NCH2PCy2)2C6H4 (14)................ 64 4.4.7 GeFe2(C5H5)2(NCH2PCy2)2C6H4 (15)................. 64 4.5 References . 65 4.6 Figures, Schemes, and Tables . 66 5 Reactivity with Carbon Dioxide 90 5.1 Abstract . 90 5.2 Introduction . 90 5.3 Results and Discussion . 92 5.4 Experimental Section . 95 5.4.1 Synthetic Materials and Methods . 95 5.4.2 Si2Pd2((NCH2PCy2)2C6H4)2 (16)................... 96 5.4.3 Si2Pd2CO2((NCH2PCy2)2C6H4)2 (17)................. 96 5.4.4 Si2Pd2CO2((NCH2PCy2)2C6H4)2 (17)................. 97 5.4.5 Si2Pd2O((NCH2PCy2)2C6H4)2 (18).................. 98 5.5 References . 100 5.6 Figures, Schemes, and Tables . 101 x List of Figures 1.1 Singlet VS Triplet Carbene . .2 1.2 Examples of NHC's in Main Group Chemistry . .3 1.3 1st and 2nd Generation Grubbs Catalysts . .4 1.4 First Stable Silylene . .5 1.5 Four Types of Silylenes . .5 1.6 Demonstration of Si and Ge tuning Ability for C-H Borylation of Arenes . .6 1.7 Nickel pre-catalyst for C-C cross-coupling reactions . .7 2.1 Phosphine Pincer Ligands . 11 2.2 Potential Routes to a Metal Silylene Complex . 14 1 2.3 HNMR of 1 in C6D6, 300MHz . 19 31 2.4 PNMR of 1 in C6D6, 300MHz . 20 29 2.5 SifHg HSQC NMR of 1 in C6D6, 300MHz . 21 1 2.6 HNMR of 2 in C6D6, 300MHz . 22 31 2.7 PNMR of 2 in C6D6, 300MHz . 23 29 2.8 SifHg HSQC NMR of 2 in C6D6, 300MHz . 24 1 2.9 HNMR of 3 in C6D6, 300MHz . 25 31 2.10 PNMR of 3 in C6D6, 300MHz . 26 1 2.11 HNMR of 4 in C6D6, 300MHz . 27 31 2.12 PNMR of 4 in C6D6, 300MHz . 28 1 2.13 HNMR of 5 in C6D6, 600MHz . 29 31 2.14 PNMR of 5 in C6D6, 600MHz . 30 13 2.15 CNMR of 5 in C6D6, 600MHz . 31 2.16 Thermal ellipsoid plot at 50% probability of the germylene complex 4. Or- ange, blue, teal, and grey ellipsoids correspond to phosphine, nitrogen, ger- manium, and carbon atoms, respectively. Hydrogen atoms bonded to carbon have been omitted for clarity. 33 3.1 Routes to Group 10 Metal Silyl Complexes . 34 3.2 Unambiguous H atom bridging metal and silicon . 35 1 3.3 HNMR of 6 in C6D6, 600MHz. 44 31 3.4 PNMR of 6 in C6D6, 600MHz. 45 xi 13 3.5 CNMR of 6 in C6D6, 300MHz. 46 29 3.6 SiNMR of 6 in C6D6, 600MHz. 47 1 3.7 HNMR of 8 in C6D6, 400MHz. 48 31 3.8 PNMR of 8 in C6D6, 300MHz. 49 13 3.9 CNMR of 8 in C6D6, 600MHz. Impurities are denoted with a * . 50 29 3.10 SiNMR of 8 in C6D6, 600MHz. 51 31 3.11 PNMR of 9 in C6D6, 600MHz. 52 29 3.12 SiNMR of 9 in C6D6, 600MHz. 53 4.1 Family of Bimetallic Germanium Complexes . 55 4.2 Family of Silyl and Germanyl Carbaonyl Complexes .
Details
-
File Typepdf
-
Upload Time-
-
Content LanguagesEnglish
-
Upload UserAnonymous/Not logged-in
-
File Pages129 Page
-
File Size-