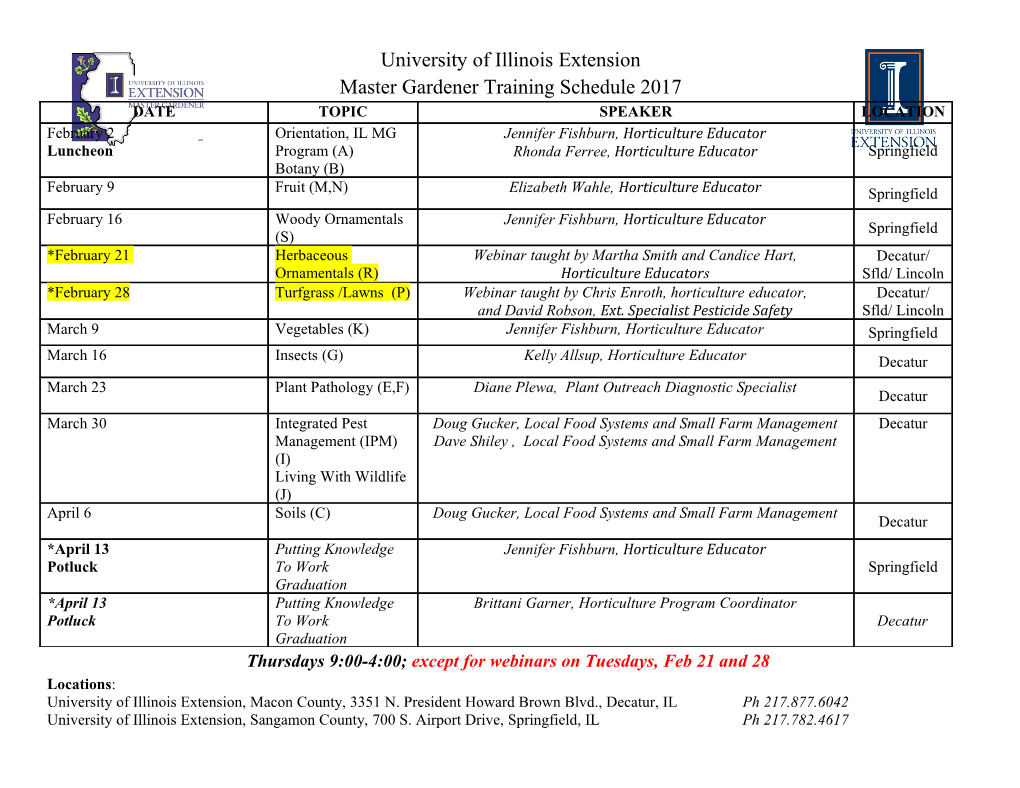
Transactions on the Built Environment vol 14, © 1995 WIT Press, www.witpress.com, ISSN 1743-3509 Liquefaction potential of silty soils D. Erteif, M.H. Maher*> "Lockwood-Singh and Associates, 1944 Cotner Avenue, ^Department of Civil and Environmental Engineering, Rutgers University, Piscataway NJ 08855-0909, USA Abstract Cyclic stress controlled tests are performed to investigate the degree of liquefaction of sand containing cohesionless and low plasticity fines such as those found in tailing materials. The experimental parameters varied consists of sand void ratio, silt content, and plasticity of silt. The failure is defined as 5% and 10% peak to peak axial strain since the pore pressure does not fully developed and stopps building up when it reaches a value equal to 90-95% of the initial confining stress. 1 Introduction Liquefaction of soils is a complex problem on which a great deal of experimental and numerical research has been done (NRC 8). Much of the research done involved liquefaction of sands. Hence, soils containing some fines are frequently encountered rather than clean sands, especially in alluvial or reclaimed deposits where soil liquefaction becomes extremely important for evaluating the stability of the ground during earthquakes. The phenomenon of liquefaction is considered to be the main cause of slope failures in saturated deposits of fine silty sands. Many natural and artificial soil deposits contain silty layers and the permeability of the soil depends on the amount of silt percent. For example, Mid-Chiba Earthquake shook the area of Tokyo Bay in 1980 with a magnitude of 6.1. Records of time change of pore pressures Transactions on the Built Environment vol 14, © 1995 WIT Press, www.witpress.com, ISSN 1743-3509 164 Soil Dynamics and Earthquake Engineering indicated that there were differences in the rate of pore pressure dissipation in different layers which contained different amount of fines (Ishihara^). The pore pressure dissipated faster in the layers with 12% fines compared to layers with higher percentage of fines. This paper describes the results of an experimental study on the effect of fine content on pore pressure generation in sand using laboratory stress controlled undrained cyclic triaxial tests. Detailed description of the experimental procedure and the results of the tests are tabulated at (Erten % ). 2 Testing Program and Procedure Cyclic stress controlled tests were run by a recently developed automated testing system. Loading is controlled by a closed-loop feedback scheme capable of performing stress controlled tests in the standard triaxial environment. Loading conditions are microprocessor controlled and electronic instrumentation is used for data acquisition and processing (Chan \ Li 4 ). The cyclic tests were run to a double amplitude axial strain of 15%. All the tests were performed at a loading rate of one cycle per minute to ensure equalized pore pressure readings. The properties of the soils tested are given in Table 1 (a) and (b). The grain size distribution and hydrometer analysis for the tested soils are shown in Fig.l. The method of undercompaction was used for specimen preparation. The control parameter was chosen as void ratio because different amounts of silt were added to the sand by weight such that the maximum and minimum void ratios of each sample was different for every placement condition. The specimens were prepared in 10 layers using the undercompaction wet tamping method in order to achieve a more uniform density. Details of undercompaction method is given by Ladd 3. The specimens were saturated by "vacuum procedure" (Rad ?) until they were fully saturated. Saturation was completed by the help of backpressure saturation. The pore pressure and cell pressure were increased in increments up to 345 kPa. After the saturation process was completed, the specimens were isotropically Transactions on the Built Environment vol 14, © 1995 WIT Press, www.witpress.com, ISSN 1743-3509 Soil Dynamics and Earthquake Engineering 165 Table 1. The Index Properties Of the Soils Used (a) Sand and Non-Plastic Silt, (b) Silty Clay Index Properties Ot;towa Sand(C-190) Sil-Co-Sil 125 Composition Ground Silk;a Ground Silica Specific Gravity 2.65 2.65 &max 0.78 1.35 &min 0.5 0.5 Cu 1 7.5 DIG 0.50 0.003 D50 0.6 0.017 090 0.76 0.068 Liquid Limit 22 22 Plastic Limit NP NP Plasticity Index NP NP Particle Shape Rounded Roundeid Sphericity 0.85 0.85 Unified Soil Classification SP ML Index Properties Silty Clay Specific Gravity 262 Liquid Limit 35 Plastic Limit 25 Plasticity Index 10 Optimum Moisture, % 26 Unified Soil Classification ML Maximum Density, pcf 104 Pass #4, % 100 Pass #200, % 90 SAND SILT CLAY 100 0.1 0.01 0.001 0.0001 Grain Diameter, mm Figure 1: Grain Size Distribution of the Tested Soils Transactions on the Built Environment vol 14, © 1995 WIT Press, www.witpress.com, ISSN 1743-3509 166 Soil Dynamics and Earthquake Engineering consolidated. Effective consolidation stress of 98 kPa. was used for all tests. Axial strain, volume changes, and pore pressure changes were recorded. 3 Results and Discussion Fig.2 shows the cyclic stress ratio vs. number of cycles on loosely constituted sand specimen. As the cyclic stress ratio decreases, the number of cycles required to induce 2.5, 5.0, 10.0 % strain and initial liquefaction almost collapse on each other. This indicates that the development of initial liquefaction occurs following sudden increases of cyclic strains even at the same cycles. In medium condition, as shown in Fig.3 the trends seems more or less the same but with higher stress ratios. The initial liquefaction immediately follows 10% strain faster than in loose condition. For specimens prepared by addition of 10% non-plastic silt in both loose and medium conditions, the cyclic strength curve is rather steep. For medium condition, the curves showed the same trend as loose sand and initial liquefaction occurred after a few cycles following 10% strain. For lower stress ratios, initiation of 10% cyclic strain was immediately followed by initial liquefaction (Figs. 4 & 5). The results of cyclic tests on sands with 10% low-plasticity fines are plotted in Fig. 6 in terms of the cyclic stress ratio versus the number of cycles. It may be observed that addition of both non-plastic and low- plasticity fines accelerated the pore pressure generation (Figs. 7 and 8). This effect is more pronounced with the addition of non-plastic fines. The cyclic resistance curves showing cyclic stress ratio, <Jj I 2cr^, to cause liquefaction (5.0% double amplitude criterion in 20 stress cycles) versus void ratio are displayed in Fig. 9. As silt content increases, the resistance curves shift towards the lower void ratios. The cyclic resistance at a given void ratio decreases with increases in silt content. It can be said that as the amount of silt increases, the soil becomes more susceptible to liquefaction and the effect of changes in cyclic stress ratio is more pronounced. The increase in cyclic resistance for the same decrease in void ratio is not same for all the specimens. As the silt content increases up to 30%, the specimen is more effected from void ratio changes. Transactions on the Built Environment vol 14, © 1995 WIT Press, www.witpress.com, ISSN 1743-3509 Soil Dynamics and Earthquake Engineering 167 0.45 Loose Condition • Initial Liquefaction Ottowa Sand 20-30 X 2.5% strain 0.4 0=98 kPa. O 5.0% strain A 10% strain 035 0.3 0.25 X29 0.2 10 "100 Number of Cycles, N Figure 2: Cyclic Stress Ratio vs. Number of Cycles Not Failed at the End of 1000th Cycle at S=0.2 Medium Condition • Initial Liquefaction - Ottowa Sand 20-30 X 2.5% strain 0=98 kPa. O 5.0% strain 4- 10% strain 0.35 0.3 0.25 1 10 100 Number of Cycles, N Figure 3: Cyclic Stress Ratio vs. Number of Cycles 0.45 : 'e= 0.64-6.60 ' '; Content of fines: 10% o 0.4 L Type of fines: Non-plastic J /2 a , ! # 2.5% strain ! 0.35 A 5.0% strain - Ratio : o 10.0% strain ; s 0.3 Stres c 0.25 Cycli 0.2 ^ e ^ 10 iwr 1000 Number of Cycles, N Figure 4: Cyclic Stress Ratio vs. Number of Cycles Transactions on the Built Environment vol 14, © 1995 WIT Press, www.witpress.com, ISSN 1743-3509 168 Soil Dynamics and Earthquake Engineering 0.4 I e= 0.54-0.58 '. Content of fines : 1 0% ; a 0.35 1 Type of fines: Non-plastic /2 r c , 0.3 Ratio s 0.25 '- 0 -_ Stres c i >> 0.2 - • 2.5% strain o - U A 5.0% strain : O 10.0% strain 0.15 10 100 Number of Cycles, N Figure 5: Cyclic Stress Ratio vs. Number of Cycles 0.45 e=0.52-0.58 Content of fines: 10% § 0.4 Type of fines : Low-plasticity •g 0.35 oJ g 0.3 ^ 0.25 10 100 1000 Number of Cycles to Liquefaction, N Figure 6: Cyclic Stress Ratio vs. Number of Cycles 0.4 : ' e=o! 54-0.58 ' I Content of fines :20% 0.35 1 Type of fines: Non-plastic - < • 2.5% strain ' A 5.0% strain - o 0.3 g>- O o 10.0% strain - 04 0.25 oo 1 0 -_ 0.15 10 100 1000 Number of Cycles, N Figure 7: Cyclic Stress Ratio vs. Number of Cycles Transactions on the Built Environment vol 14, © 1995 WIT Press, www.witpress.com, ISSN 1743-3509 Soil Dynamics and Earthquake Engineering 169 e= 0.52-0.58 Content of fines:30% 0" 0.35 Type of fines: Non-plastic A O •£0 0.15 10 100 Number of Cycles, N Figure 8: Cyclic Stress Ratio vs.
Details
-
File Typepdf
-
Upload Time-
-
Content LanguagesEnglish
-
Upload UserAnonymous/Not logged-in
-
File Pages9 Page
-
File Size-