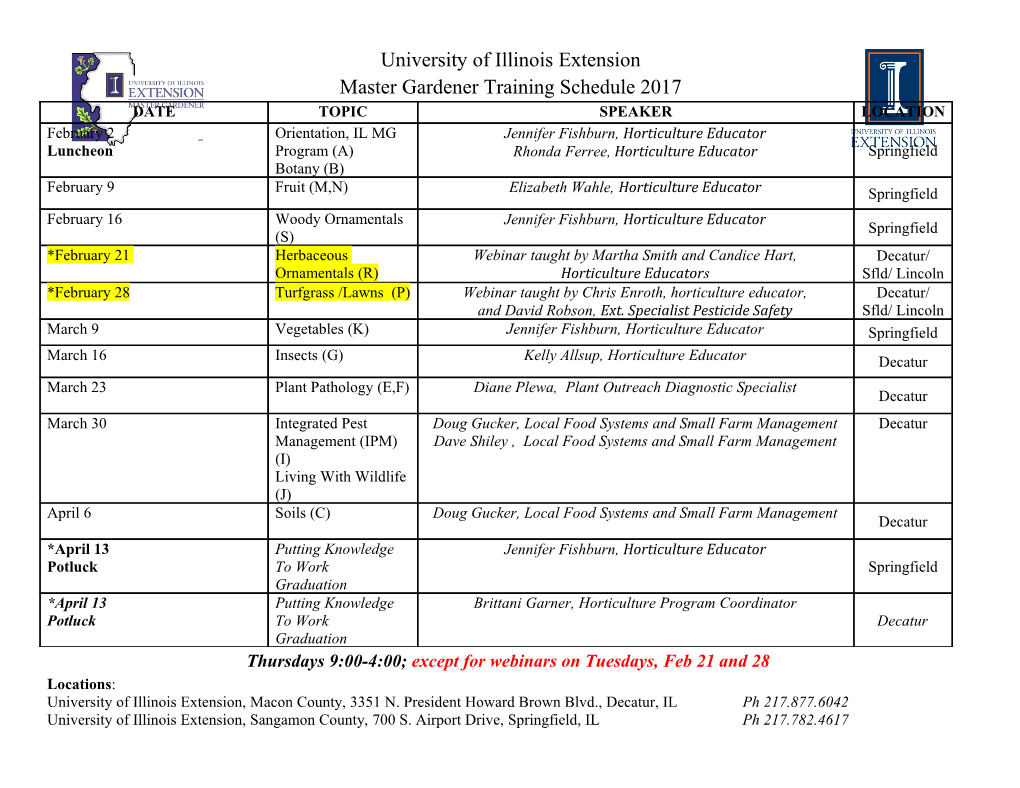
SCHOOL OF PHYSICAL SCIENCES LOW POWER, LONG DURATION ROTAMAK DISCHARGES IN ARGON B.L. JESSUP and J. TENDYS FUPH-R-180 FEBRUARY, 1982 LOW POKER, LONG DURATION ROTAMAK DISCHARGES IN ARGON B.L. Jessup and J. Tendys* School of Physical Sciences The Flinders University of South Australia Bedford Park, 5042, Australia ABSTRACT An investigation was made of a Rotamak compact torus configuration in which the rotating magnetic field used to drive the toroidal current was generated by a pulsed 6 KW R.F. oscillator. The toroidal current was measured together with the vertical component of the magnetic field both along the vertical axis and as a function of radius in the midplane of the current ring. The experimental results show that a Rotamak equilibrium, apparently stable for several milliseconds, could be obtained. Toroidal plasma currents of several hundred amps were observed with argon filling pressures around 0.2 mTon and applied vertical equilibrium fields of about 1 m Tesla. * Visiting Scholar, on attachment from the Australian Atomic Energy Commission. 1. I. INTRODUCTION The R^tamak concept, which uses a rotating magnetic field to drive the steady toroidal current in a compact torus device, has been studied experimentally using high power (10 Mm"), short time (60 usee) rotating magnetic field pulses produced by line generators (W.H. HUGRASS, I.R. JONES, K.F. McKENNA, N.G.R. PHILLIPS, R.G. STORER, and H. TUCZEK, 1980). It is of considerable interest to extend this study to longer lived Rotamak plasmas- The objective of these experiments was to verity the maintenance of an equilibrium configuration of milli-second lifetime. In this report we describe the construction of a Rotamak device which uses a low power (6 KW) C.W. oscillator. Due to heating of the circuit components used to couple the RF powe- to the rotating field coils, the rotating magnetic field pulse was rarely allowed to exceed 12 ms. The Rotaaak equilibrium field configuration was studied during this time interval using magnetic probes and a current loop, which was used to monitor the total toroidal current. II. EXPERIMENTAL APPARATUS 2.1 Vacuum System The spherical vacuum vessel was made of pyrex glass with a 0.135 m radius, and had two diametrically opposed 0.05 • diameter ports, Fig. 1. One port was used for the pressure gauges, while the other was used for pumping and gas input. When experiments were not in progress the vacuum vessel was maintained at a base pressure of 3 x 10' Torr by means of a 6" alcatel oil diffusion pump fitted with a Balzer water-cooled baffle and backed by a two stage rotary pump. During the experiments argon gas was allowed to enter via a 4 an I.D. pyrex tube attached to one of the ports. •> The gas was permitted to flow continuously into the system maintaining an equilibrium pressure, controlled by a needle valve. The gas pressure in the vessel was monitored between plasma discharges by a Cooke ionization gauge. 2.2 Rotating Magnetic Field Generator The rotating magnetic field power source was a 6 KW Class C oscillator which was tuned to 0.845 MHz. The oscillator tank inductance consisted of two sections connected in series. One section was modified to permit the powsr to be taken out by inductive coupling. This modified part of the tank circuit inductance was made from 0.01m O.D. copper tube formed into three sections of three turns each with a diameter of 0.13 m, Fig. 2. The two secondary coils each 0.08 m in length, made from 11 turns of 0.005 m O.D. copper tube, had a diameter of 0.13 m. and an inductance of 30 WH each. Each secondary coil was supported on a hinge in one of the two gaps in the modified tank inductor to permit the coupling with the primary to be varied. A variable capacitor was connected in series with each secondary coil and adjusted so that the series resonance frequency of the secondary circuit matched that of the tank circuit. The coupling of the secondaries was then adjusted until maximum power was fed into each of the two 50(2 output cables. During the adjustment both cables were terminated by 50ft resistive loads. One of the two output cables had an additional 60 m length of cable added to it which delayed the current in that arm by 0.3 us to give a 90° phase shift between the currents in the two outputs. It should be noted that the use of a delay line to obtain the required current phase shift for the generator of the rotating magnetic field presented a problem. When the length of a cable is exactly an odd number of quarter wave lengths, 3. as was the case with the delay cable used in these experiments, then the input impedance Z of the cable is given by Z 2 where ZQ is the cable iapedance and Z, is the load impedance (F.E. TERMAN, 1943). Accordingly if Z,, which is nominally 50ft, gains a small capacitive or inductive component then Z will no longer be SOQ but will have a large reactive component. The load coils were connected to the 50ft cables by matching circuits, Fig. 2. The matching conditions are given by 2 Cj = l/(w LL - uwi0RL - R^') Ll s Cl RL2/(tt)2LL Cl " 1} * (u2LL Cl " 1W<u2ci) where R, and L, are the effective resistance and inductance of the load respectively. Due to the delay cable small mismatches caused large changes in the reactive part of the load at the tank coil; consequently the oscillator experienced large changes in its load. This caused shifts of about 1% in the operating frequency. This interaction was reduced by connecting a 100(2 resistor to ground at the input to the delay cable. Thus the adjustment of L. and C. was made easier at the expense of some of the output power from the oscillator. 2.3 Rotating Field Coils The rotating field coils fixed to the vessel consisted of two orthogonal Helmholtz coils. Each Helaholtz coil was made from 0.005 m O.D. copper tube formed into two coils of 3 turns of diaaeter of 0.27 m. The vacuum inductance of each Helaholtz coil was 12 yH. Although the two Helaholtz coils were nominally orthogonal there was 4. a mutual inductance of about 80 nH between thea. This had to be eliminated so that the two impedance aatching circuits could be adjusted independently. This was achieved by coupling the two circuits with a small variable mutual inductor formed by two loops with an adjustable separation. By choosing the sign of this mutual inductance to be opposite to that already present the net coupling could be made very small. The presence of pla'jaa changes the impedance of the rotating field coils; in practice the values of L, and Cj in the impedance aatching circuit were adjusted with a plasaa present in the vessel. A Pearson current transformer was used to measure the input current to the matching circuit and a potential divider the voltage input. The values of L. and C. were then varied until the input resistance was close to the 50Q of the cables. 2.4 Equilibrium Vertical field An externally imposed vertical field is required to maintain equilibrium. In this experiment each of the two vertical field coils were constructed from 133 turns of 1 am O.D. copper wire wound on circular formers. Each coil had a mean diameter of 0.35 m and an inductance of 10 mH. The coils were positioned equal distances above and below the discharge vessel with a coaxial separation of 0.5 a. Current was fed to the coils from a capacitor bank consisting of four 300 uf capacitors connected in parallel. The bank, fired by triggering an SCR, gave an asyaaetrical current pulse with a first half period of 18 as. At the peak of the pulse the current reaained constant for 2 as to better than 10%. The systea was designed to supply a vertical field strength of 10 aTesla at the centre of the discharge vessel. However, it was found experiaentally that fields of only about 1 mTesla were needed. The vertical field was applied in the negative z-direction. s. 2.5 Pre-ionization At pressures of less than 1 aTorr of argon the rotating magnetic field was insufficient to break down the gas electrically. To overcoae this problem the gas was very weakly preionized by creating an R.F. discharge in the vessel. The mechanism by which the R.F. discharge was created was not well understood and the final experiaental configuration of exciting coils discussed below was obtained by a process of trial and error. A signal generator was used to drive a 300 watt broad-band power amplifier. The output of the amplifier was connected to two coil structures made of 2 am O.D. copper wire, Fig. 1. The first of the two structures was a four turn coil of diameter 0.15 a. The second consisted of two short solenoids, 6 and 4 turps respectively, wound on the neck of the vessel and separated by 0.06 a. All the coils were electrically connected in series. In addition the aluainiua end plates fitted to the discharge vessel (see Fig. 1) were electrically connected to the output cable of the amplifier so that a tiae varying potential was generated along the length of the vessel. This configuration, although quite arbitary, proved successful in preionizing argon at pressures down to 0.1 aTorr.
Details
-
File Typepdf
-
Upload Time-
-
Content LanguagesEnglish
-
Upload UserAnonymous/Not logged-in
-
File Pages40 Page
-
File Size-