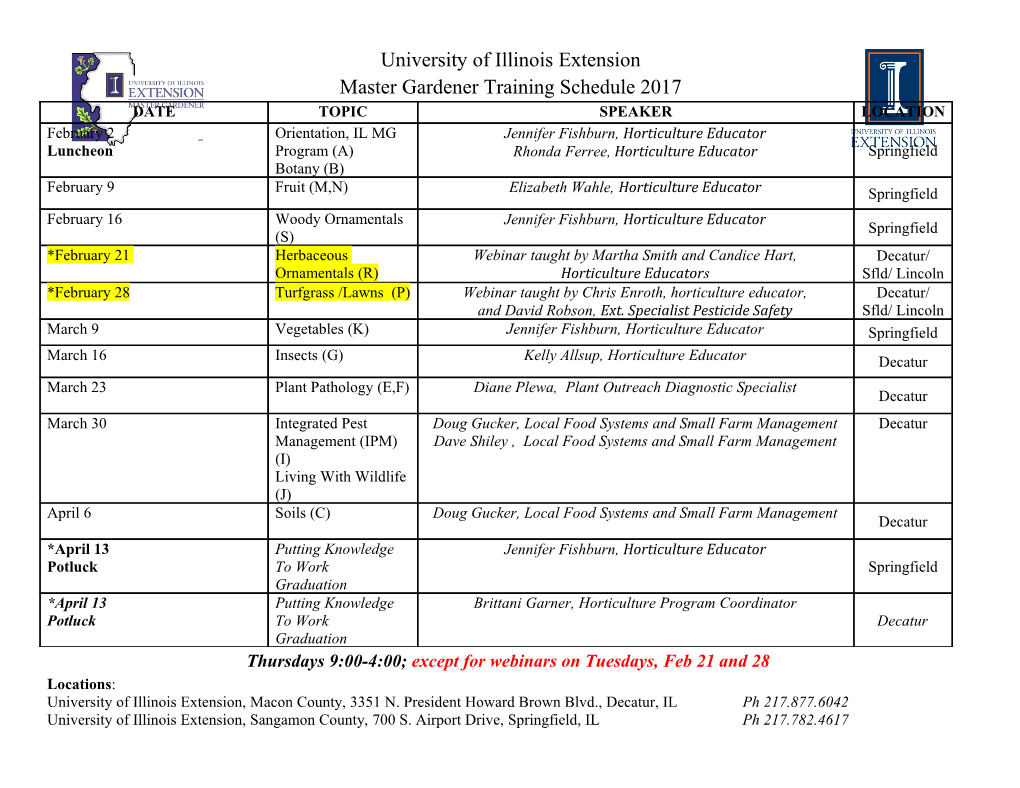
Available online at www.sciencedirect.com ScienceDirect Controlling entropy to tune the functions of intrinsically disordered regions 1 1 Tilman Flock , Robert J Weatheritt , Natasha S Latysheva and M Madan Babu Intrinsically disordered regions (IDRs) are fundamental units of extensive functional regions within polypeptide seg- protein function and regulation. Despite their inability to form a ments that do not form stable tertiary structure. These unique stable tertiary structure in isolation, many IDRs adopt a regions, referred to as intrinsically disordered regions defined conformation upon binding and achieve their function (IDRs), exist in over 35% of human proteins [2,3] func- through their interactions with other biomolecules. However, this tioning in all major cellular processes with significant requirement for IDR functionality seems to be at odds with the enrichment in signalling and regulation [4,5 ]. Although high entropic cost they must incur upon binding an interaction IDRs are capable of performing functions comparable to partner. How is this seeming paradox resolved? While increasing structured regions, they vary significantly in their amino the enthalpy of binding is one approach to compensate for this acid composition [6] and their biophysical properties entropic cost, growing evidence suggests that inherent features [7 ]. Together this suggests that IDRs are independent of IDRs, for instance repeating linear motifs, minimise the units of protein function, capable of achieving biological entropic cost of binding. Moreover, this control of entropic cost function in a manner that is distinct from that of struc- can be carefully modulated by a range of regulatory tured regions [1 ,7 ]. mechanisms, such as alternative splicing and post-translational modifications, which enable allosteric communication and Energy landscape of intrinsically disordered rheostat-like tuning of IDR function. In that sense, the high regions in proteins entropic cost of IDR binding can be advantageous by providing In contrast to structured domains that are primarily gov- tunability to protein function. In addition to biological regulatory erned by non-covalent tertiary contacts between second- mechanisms, modulation of entropy can also be controlled by ary structural elements, IDRs do not adopt a well-defined environmental factors, such as changes in temperature, redox- tertiary structure. Instead they are in a dynamic equi- potential and pH. These principles are extensively exploited by a librium between different sets of conformational states number of organisms, including pathogens. They can also be [8] (Figure 1). The probability of finding an IDR in a utilised in bioengineering, synthetic biology and in certain state within this conformational (or statistical) pharmaceutical applications such as increasing bioavailability of ensemble is related to its conformational free energy protein therapeutics. landscape [7 ,8]. The native state of IDRs is therefore Addresses best described in terms of a relatively flat but rugged MRC Laboratory of Molecular Biology, Francis Crick Avenue, Cambridge energy landscape with numerous local energy minima CB2 0QH, UK separated by low energetic barriers [9,10]. This strongly contrasts with the energy landscape of a structured Corresponding authors: Weatheritt, Robert J (rweather@mrc- lmb.cam.ac.uk), Babu, M Madan ([email protected]) domain, which exhibits a well-defined minimum energy 1 These authors contributed equally to this work. state [7 ], resulting in one or a few clearly favoured conformational states (Figure 1). Because of their flat Current Opinion in Structural Biology 2014, 26:62–72 energy landscape, IDRs can exist in multiple energeti- cally similar but conformationally different states. In This review comes from a themed issue on Sequences and topology biophysical terms, this means that IDRs exhibit high Edited by L Aravind and Christine Orengo conformational entropy (Figure 1). When participating in binding events with other biomolecules, IDRs must pay an entropic cost due to transitioning from a large set of http://dx.doi.org/10.1016/j.sbi.2014.05.007 conformations in the free form to a more restricted 0959-440X/# 2014 Elsevier Ltd. All rights reserved. arrangement in the bound form [11]. How do IDRs exhibit functional properties when the potential entropic penalty of engaging in functionality is likely to be high? Recent studies have suggested that the large entropy loss due to a disorder-to-order transition upon binding can be Introduction counter-balanced by a comparable gain in enthalpy of There is an increasing appreciation that structure is not an binding [12–14]. In many cases, this has been suggested essential prerequisite for many kinds of protein function to result in an interaction that is characterised by high [1 ]. An ever-growing number of studies have identified specificity but low affinity of binding. IDRs achieve this Current Opinion in Structural Biology 2014, 26:62–72 www.sciencedirect.com Exploiting entropy for biomolecular interaction Flock et al. 63 Figure 1 Native Disordered State Preferred Conformations Ordered State Free Energy Free Energy Free Energy Free Energy Landscape Ordered Disordered Statistical Ensemble Single conformation dominates Many different conformations sampled Current Opinion in Structural Biology The free energy landscape and conformational ensembles of IDRs can be dynamically tuned. The free energy landscape schematically represents any possible conformation (x–y axes) a protein can adopt, as well as its respective free energy (z-axis) ( free energy landscape, upper row), and thus describes the probability of finding any particular conformation in an ensemble of conformational states (statistical ensemble, lower row). Whereas structured proteins have energy landscapes with a clearly preferred conformation (global minimum, right panel), disordered proteins in their native form have a rugged, flat energy landscape allowing multiple conformations to be thermodynamically favourable and to be found in the statistical ensemble (left panel). Several factors can modulate the free energy landscape of IDRs and restrict the statistical ensemble to fewer, more defined conformations (middle panel). As conformational entropy decreases, so does the number of observed conformational states. In the most ordered state, the disordered protein landscape turns into the funnel-shaped landscape of structured proteins, shifting the statistical conformational ensemble towards a predominant conformation (right panel). This fine-tuning of the statistical ensemble of IDRs enables specific functions and tunability. by having an extensive interaction interface capable of IDRs upon binding as a disadvantage, it may be regarded participating in a large number of weak non-covalent as highly advantageous in many cases. This is because contacts [12,14]. However, there are many examples of modulation of the entropic cost enables IDRs to dyna- IDRs binding with a limited interaction surface area and/ mically change their energy landscapes. This results in or in their disordered state [15 ,16]. So what other the creation of a wide range of regulatory functions that additional mechanisms allow IDRs to overcome the can be actively and intricately tuned by intrinsic and entropic penalty of binding and become functional? In extrinsic factors. this article, we discuss the role of entropic contribution to free energy (DG = DH À TDS; see Box 1) in IDR function How inherent features of IDRs permit and highlight that minimising the relative entropic cost exploitation of entropy for functionality upon binding is a prerequisite for functions mediated by What are the potential ways to counter-balance the several IDRs. We further discuss that rather than con- entropic cost of binding of IDRs and modulate their sidering the potentially large entropic cost incurred by free energy landscape? Deriving from the definition of www.sciencedirect.com Current Opinion in Structural Biology 2014, 26:62–72 64 Sequences and topology Box 1 Thermodynamic properties in the context of polypeptides conformational entropy at the motif interface is limited. and their ligands. Since many motifs do not form secondary structure Relative enthalpy (DH) describes the change in internal energy of elements upon binding, the region mediating the the system as well as the impact on its surrounding environment. In interaction remains flexible, permitting extensive back- protein folding, this is mainly determined by electrostatic interactions bone-mediated hydrogen bonds [18 ,19] and thereby and non-covalent bonds between amino acids within secondary maximising the potential enthalpy of binding within structural elements of structured domains. Relative entropy (DS) is a measure of the change in randomness the limited interface of the motif. When motifs do have (number of distinct states) of a system. In biological systems, the main secondary structure, it is typically preformed and rigid in factors contributing to entropy are the degree of conformational the native state (e.g. polyproline stretches), minimising freedom of the polypeptide chain, entropy of complex formation (e.g. the loss of conformational entropy upon binding [15 ,20]. binding of ligand to the polypeptide), and entropy changes due to the Secondly, the interaction partners of motifs tend to have rearrangement of surrounding water (solvent) molecules. Configurational entropy (DSconfig) of a protein is related to the rigid interfaces, which ensure that
Details
-
File Typepdf
-
Upload Time-
-
Content LanguagesEnglish
-
Upload UserAnonymous/Not logged-in
-
File Pages11 Page
-
File Size-